Chemical Change: Identify and apply knowledge of electrochemical cells
Unit 1: Electrochemical Cells
Emma Harrage
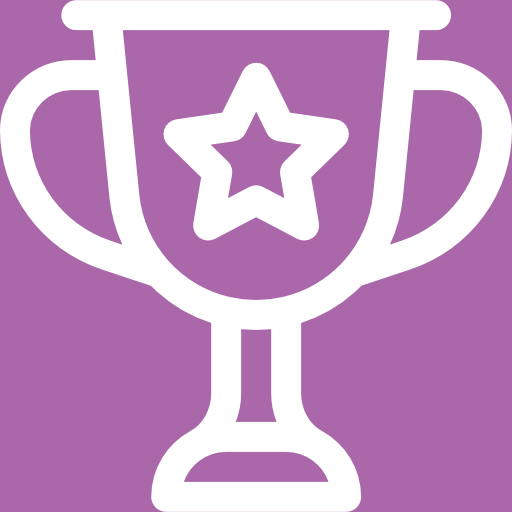
Unit outcomes
By the end of this unit you will be able to:
- Define oxidation, reduction, cathode, anode, and electron transfer.
- Write oxidation and reduction half reactions.
- Describe the purpose of a sacrificial anode and corrosion of metals and write reactions down to illustrate.
- Describe an electrochemical cell and identify examples in everyday life/industry.
- Draw and label an electrochemical cell and determine cell potential and compare with cells used in everyday life (Li, Cd, Pb- cells).
What you should know
Before you start this unit, make sure you:
- Can balance chemical equations. Refer to level 3 subject outcome 6.2 unit 1 if you need help with this.
- Understand oxidation numbers. Refer to level 3 subject outcome 5.2 unit 3 if you need help with this.
- Understand that there are different types of chemical reactions. Refer to level 3 subject outcome 6.3 unit 1 if you need help with this.
Introduction
Parts of the text in this unit were sourced from Siyavula Physical Science Gr 12 Learner’s Book, Chapter 13, released under a CC-BY licence.
In this unit you will learn about electrolysis which is what happens inside an electrolytic cell. Electrolysis is a process where electricity is used to make a chemical change happen. The most common usage is for decomposition. Sodium chloride is broken down by electrolysis to obtain sodium and chlorine. Sodium chloride must be melted until it is liquid and then the [latex]\scriptsize \displaystyle \text{N}{{\text{a}}^{\text{+}}}[/latex] ions and the [latex]\scriptsize \displaystyle \text{C}{{\text{l}}^{\text{-}}}[/latex] can move to the oppositely charge anode.
There are two types of electrochemical cell – electrolytic in which electricity is used for decomposition, and galvanic which are used to make electricity, both involving redox reactions. Electrochemical cells use metallic electrodes in an electrolyte solution to generate a current and are called galvanic cells. Batteries usually have at least two of these types of cells.
Redox reactions
Redox reactions involve the exchange of electrons. One species loses electrons and becomes more positive, while the other species gains electrons and becomes more negative. To decide if a redox reaction has occurred, we look at the charge of the atoms, ions or molecules involved. If one of them has become more positive and the other one has become more negative, then a redox reaction has occurred. For example, sodium metal is oxidised to form sodium oxide and sometimes sodium peroxide as well. The balanced equation for this is:
[latex]\scriptsize \displaystyle \text{4Na+}{{\text{O}}_{\text{2}}}\to \text{2N}{{\text{a}}_{\text{2}}}\text{O}[/latex]
In the above reaction sodium and oxygen are both neutral and so have no charge. In the products however, the sodium atom has a charge of [latex]\scriptsize \displaystyle {{1}^{+}}[/latex] and the oxygen atom has a charge of [latex]\scriptsize \displaystyle {{2}^{-}}[/latex]. This tells us that the sodium has lost electrons and the oxygen has gained electrons. Since one species has become more positive and one more negative, we can conclude that a redox reaction has occurred. We could also say that electrons have been transferred from one species to the other.
Redox is short for and .
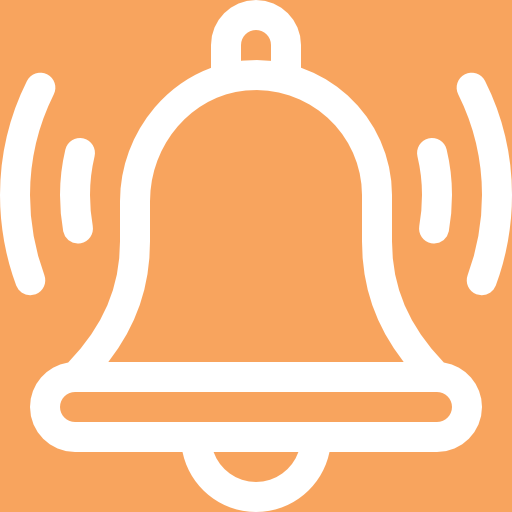
Take note!
Remember that:
Oxidation involves a loss of electrons
Reduction involves a gain of electrons.
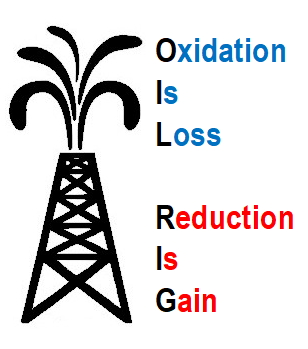
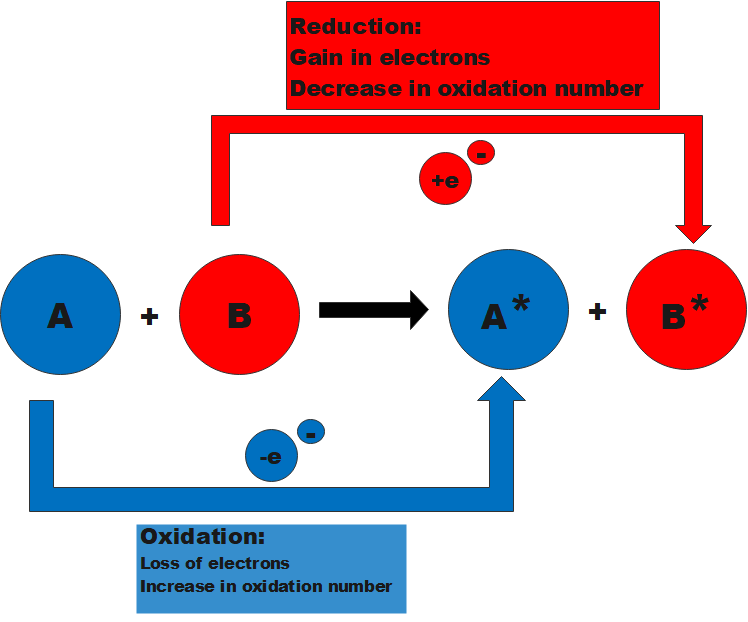
An element or compound that loses electrons is oxidised.
e.g. [latex]\scriptsize \displaystyle \text{Z}{{\text{n}}_{{\left( \text{s} \right)}}}\to \text{Z}{{\text{n}}^{{\text{2+}}}}_{{\left( {\text{aq}} \right)\text{ }}}\text{+ 2}{{\text{e}}^{\text{-}}}[/latex]
As it loses electrons it gives them away to another element or compound and the element or compound it gives the electrons to is reduced. This makes the compound or element which loses electrons a .
An element or compound that gains electrons is reduced.
e.g. [latex]\scriptsize \displaystyle \text{C}{{\text{u}}^{{\text{2+}}}}_{{\left( {\text{aq}} \right)}}\text{ + 2}{{\text{e}}^{\text{-}}}\to \text{C}{{\text{u}}_{{\left( \text{s} \right)}}}[/latex]
As it gains electrons it takes them away from another element or compound and the element or compound it takes them from is oxidised. This makes the compound or element which gains electrons an .
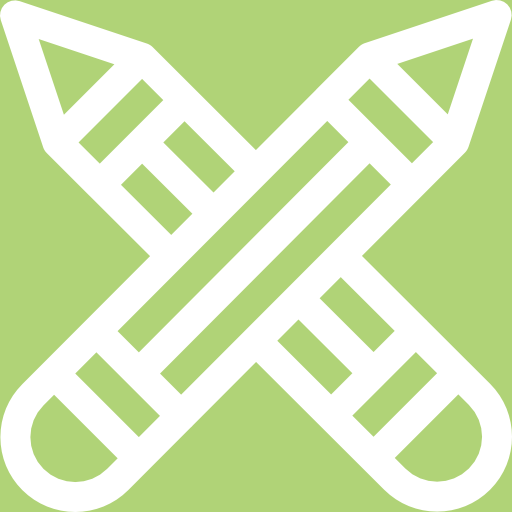
Exercise 1.1
- Define the following terms:
- oxidation
- reduction
- oxidising agent
- reducing agent
- In each of the following reactions say whether the reactant iron species ([latex]\scriptsize \displaystyle \text{Fe, F}{{\text{e}}^{{\text{2+}}}}\text{, F}{{\text{e}}^{{\text{3+}}}}[/latex]) is oxidised or reduced.
- [latex]\scriptsize \displaystyle \text{F}{{\text{e}}_{{\left( \text{s} \right)}}}\to \text{F}{{\text{e}}^{{\text{2+}}}}_{{\left( {\text{aq}} \right)}}\text{+2}{{\text{e}}^{\text{-}}}[/latex]
- [latex]\scriptsize \displaystyle \text{F}{{\text{e}}_{\text{2}}}{{\text{O}}_{{\text{3}\left( \text{s} \right)}}}\text{+2A}{{\text{l}}_{{\left( \text{s} \right)}}}~\to \text{ A}{{\text{l}}_{\text{2}}}{{\text{O}}_{{\text{3}\left( \text{s} \right)}}}\text{+2F}{{\text{e}}_{{\left( \text{s} \right)}}}[/latex]
The full solutions are at the end of the unit.
Writing redox reactions using half-reactions
Remember that oxidation and reduction occur simultaneously in a redox reaction. The reactions taking place in electrochemical cells are redox reactions. Two questions should be asked to determine if a reaction is a redox reaction:
- Is there an atom or ion being oxidised?
- Is there an atom or ion being reduced?
If the answer to both of these questions is yes, then the reaction is a redox reaction. For example, this reaction is a redox reaction:
[latex]\scriptsize \displaystyle \text{2Fe}_{{\left( {\text{aq}} \right)}}^{{\text{3+}}}\text{+Sn}_{{\left( {\text{aq}} \right)}}^{{\text{2+}}}\to \text{ 2Fe}_{{\left( {\text{aq}} \right)}}^{{\text{2+}}}\text{+Sn}_{{\left( {\text{aq}} \right)}}^{{\text{4+}}}[/latex]
You can write a redox reaction as two half-reactions, one showing the reduction process, and one showing the oxidation process. [latex]\scriptsize \displaystyle \text{F}{{\text{e}}^{{\text{3+}}}}[/latex] is gaining an electron to become [latex]\scriptsize \displaystyle \text{F}{{\text{e}}^{{\text{2+}}}}[/latex]. Iron (III) ions are therefore being reduced and [latex]\scriptsize \text{S}{{\text{n}}^{{\text{2+}}}}[/latex] tin is the reducing agent (causing iron to be reduced).
The reduction half-reaction is: [latex]\scriptsize \displaystyle \text{Fe}_{{\left( {\text{aq}} \right)}}^{{\text{3+}}}\text{ + }{{\text{e}}^{\text{-}}}\to \text{Fe}_{{\left( {\text{aq}} \right)}}^{{\text{2+}}}[/latex]
[latex]\scriptsize \displaystyle \text{S}{{\text{n}}^{{2+}}}[/latex] is losing two electrons to become [latex]\scriptsize \displaystyle \text{S}{{\text{n}}^{{4+}}}[/latex]. Tin (II) ions are therefore being oxidised and iron (III) ions are the oxidising agent (causing [latex]\scriptsize \displaystyle \text{S}{{\text{n}}^{{4+}}}[/latex] to be oxidised).
The oxidation half-reaction is: [latex]\scriptsize \displaystyle \text{Sn}_{{_{{\left( {\text{aq}} \right)}}}}^{{\text{2+}}}\to \text{Sn}_{{\left( {\text{aq}} \right)}}^{{\text{4+}}}\text{ + 2}{{\text{e}}^{\text{-}}}[/latex]
Notice that in the overall reaction, the reduction half-reaction is multiplied by two. This is so that the number of electrons gained in the reduction half-reaction match the number of electrons lost in the oxidation half-reaction. Half-reactions can be used to balance redox reactions.
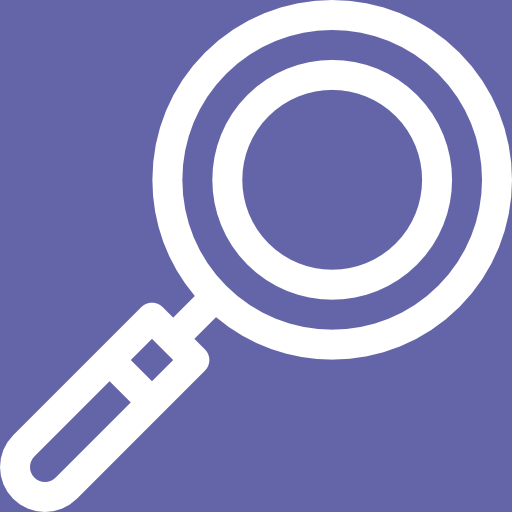
Example 1.1
Chlorine gas oxidises [latex]\scriptsize \displaystyle \text{F}{{\text{e}}^{{\text{2+}}}}[/latex]ions to:
[latex]\scriptsize \displaystyle \text{2Fe}_{{\left( {\text{aq}} \right)}}^{{\text{2+}}}\text{+ C}{{\text{l}}_{{\text{2}\left( \text{g} \right)}}}\to \text{2Fe}_{{\left( {\text{aq}} \right)}}^{{\text{3 +}}}\text{+ 2Cl}_{{\left( {\text{aq}} \right)}}^{\text{-}}[/latex] ions.
In the process, chlorine is reduced to chloride ions. Write a balanced equation for this reaction.
Solution
Step 1: Write down the unbalanced oxidation half-reaction
[latex]\scriptsize \displaystyle \text{Fe}_{{\left( {\text{aq}} \right)}}^{{\text{2+}}}\to \text{ Fe}_{{\left( {\text{aq}} \right)}}^{{\text{3+}}}[/latex]
Step 2: Balance the number of atoms on both sides of the equation
There is one iron atom on the left and one on the right, so no additional atoms need to be added. Once the atoms are balanced, check that the charges balance.
The charge on the left of the equation is [latex]\scriptsize \displaystyle +2[/latex], but the charge on the right is [latex]\scriptsize \displaystyle +3[/latex]. Therefore, one electron must be added to the right-hand side so that the charges balance.
The half-reaction is now:
[latex]\scriptsize \displaystyle \text{Fe}_{{\left( {aq} \right)}}^{{\text{2+}}}\to \text{ Fe}_{{\left( {\text{aq}} \right)}}^{{\text{3+}}}+{{\text{e}}^{-}}[/latex]
Step 3: Repeat steps 1 to 3 with the reduction half-reaction
The unbalanced reduction half-reaction is:
[latex]\scriptsize \displaystyle \text{C}{{\text{l}}_{{\text{2}\left( \text{g} \right)}}}\to \text{Cl}_{{\left( {\text{aq}} \right)}}^{\text{-}}[/latex]
The atoms don’t balance, so we need to multiply the right-hand side by two to fix this.
[latex]\scriptsize \displaystyle \text{C}{{\text{l}}_{{\text{2}\left( \text{g} \right)}}}\to 2\text{Cl}_{{\left( {\text{aq}} \right)}}^{\text{-}}[/latex]
Step 4: Two electrons must be added to the left-hand side to balance the charges
[latex]\scriptsize \displaystyle \text{C}{{\text{l}}_{{\text{2}\left( \text{g} \right)}}}\text{+2}{{\text{e}}^{\text{-}}}\to \text{2Cl}_{{\left( {\text{aq}} \right)}}^{\text{-}}[/latex]
Step 5: Compare the number of electrons in each equation
Multiply each half-reaction by a suitable number so that the number of electrons released (oxidation) is equal to the number of electrons accepted (reduction).
Oxidation half-reaction: [latex]\scriptsize \displaystyle \text{x 2: 2Fe}_{{\left( {aq} \right)}}^{{\text{2+}}}\to \text{ 2Fe}_{{\left( {\text{aq}} \right)}}^{{\text{3+}}}+2{{\text{e}}^{-}}[/latex]
Reduction half-reaction: [latex]\scriptsize \displaystyle \text{x 1: C}{{\text{l}}_{{\text{2}\left( \text{g} \right)}}}\text{+2}{{\text{e}}^{\text{-}}}\to \text{2Cl}_{{\left( {\text{aq}} \right)}}^{\text{-}}[/latex]
Step 6: Combine the two half-reactions to get a final equation for the overall reaction
Note that the electrons on each side of the redox equation will cancel each other out.
[latex]\scriptsize \displaystyle \text{2Fe}_{{\left( {\text{aq}} \right)}}^{{\text{2+}}}\text{+ C}{{\text{l}}_{{\text{2}\left( \text{g} \right)}}}\to \text{2Fe}_{{\left( {\text{aq}} \right)}}^{{\text{3+}}}\text{+ 2Cl}_{{\left( {\text{aq}} \right)}}^{\text{-}}[/latex]
Step 7: Do a final check to make sure that the equation is balanced
We check the number of atoms and the charges and find that the equation is balanced.
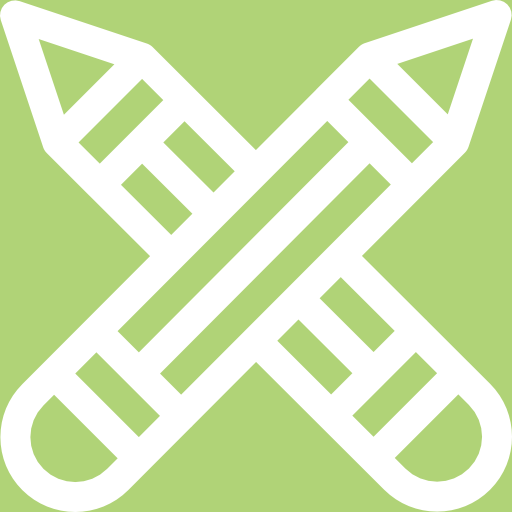
Exercise 1.2
- Balance the following redox equations in an acidic solution:
- [latex]\scriptsize \displaystyle {{\text{H}}^{+}}\text{+ M}{{\text{n}}^{{\text{2+}}}}\text{+ BiO}_{\text{3}}^{\text{-}}\to \text{MnO}_{\text{4}}^{\text{-}}\text{ + B}{{\text{i}}^{{3+}}}+{{\text{H}}_{\text{2}}}\text{O}[/latex]
- [latex]\scriptsize {{\text{H}}_{2}}\text{O + P + C}{{\text{u}}^{{\text{2+}}}}\to \text{Cu + }{{\text{H}}_{\text{2}}}\text{PO}_{\text{4}}^{\text{-}}+{{\text{H}}^{+}}[/latex]
- [latex]\scriptsize {{\text{H}}_{2}}\text{O + N}{{\text{O}}_{\text{2}}}\to \text{NO}_{\text{3}}^{\text{-}}\text{+NO + }{{\text{H}}^{+}}[/latex]
- Balance the following redox equation in a basic solution:
- [latex]\scriptsize \text{A}{{\text{l}}^{{3+}}}\text{ + }{{\text{H}}_{2}}\text{O + O}{{\text{H}}^{\text{-}}}\to \text{Al}{{\text{O}}_{\text{2}}}\text{+ }{{\text{H}}_{\text{2}}}[/latex]
- [latex]\scriptsize \text{O}{{\text{H}}^{-}}\text{ + P}{{\text{b}}^{{\text{2+}}}}\text{+ IO}_{\text{3}}^{\text{-}}\to {{\text{H}}_{\text{2}}}\text{O + Pb}{{\text{O}}_{\text{2}}}\text{+ }{{\text{I}}_{\text{2}}}[/latex]
The full solutions are at the end of the unit.
Electrolysis
Ionic substances contain charged particles called ions. For example, lead bromide contains positively charged lead ions and negatively charged bromide ions. is the process by which ionic substances are decomposed (broken down) into simpler substances when an electric current is passed through them. For electrolysis to work, the compound must contain ions. Covalent compounds cannot act as because they contain neutral atoms.
To be an electrolyte, a substance must be able to conduct electricity. To test for conductivity, include a sample of the substance in a circuit that contains a source of direct current and a bulb or an ammeter. If a current flows through the substance when it is molten or dissolved, it is an electrolyte.
The ions must be free to move, which is possible when an ionic substance is dissolved in water, or it is melted. For example, if electricity is passed through molten lead bromide, the lead bromide is broken down to form lead and bromine.
This is what happens during electrolysis:
- Positively charged ions move to the negative electrode, the , during electrolysis. They receive electrons and are reduced.
- Negatively charged ions move to the positive electrode, the , during electrolysis. They lose electrons and are oxidised. The substance that is broken down is called the electrolyte.
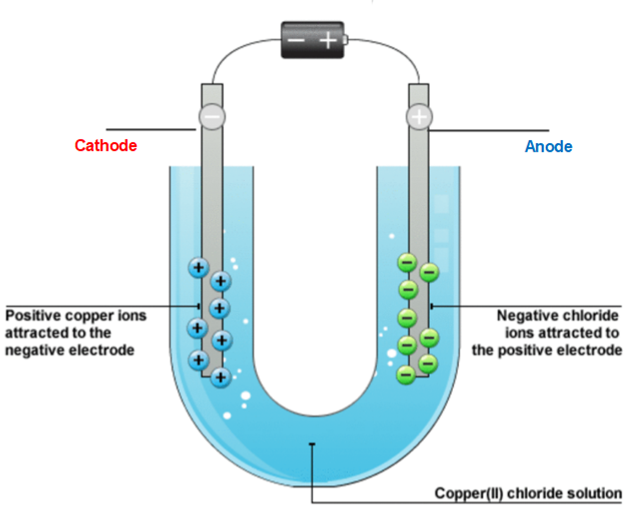
Two copper are placed in a solution of blue copper chloride and are connected to a source of electrical current. The current is turned on for a period of time. At the positive anode, copper metal is oxidised to form [latex]\scriptsize \displaystyle \text{C}{{\text{u}}^{{\text{2+}}}}[/latex] ions. This is why it appears that the copper has dissolved from the electrode. The reaction at this electrode is:
[latex]\scriptsize \text{C}{{\text{u}}_{{\text{(s)}}}}\to \text{C}{{\text{u}}^{{\text{2+}}}}_{{\text{(aq)}}}\text{+2}{{\text{e}}^{\text{-}}}[/latex]
Electrolysis of molten salts
New substances form when a molten or dissolved ionic compound conducts electricity. For example, lead bromide can be used to produce bromine and lead using the following process:
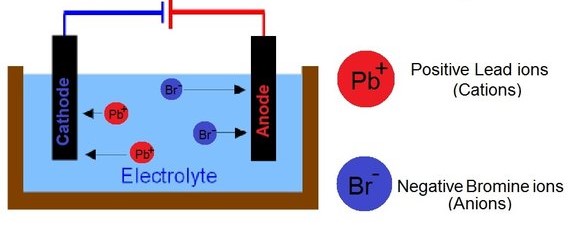
Solid lead (II) bromide is placed in a crucible and heated over a Bunsen burner until it melts. Two carbon electrodes are inserted into the molten electrolyte and a direct current is passed between them. Carbon electrodes are chosen because they have a high melting point and are inert.
The observations are summarised in the table:
Observation | Explanation |
Anode (positive) | Bubbles of brown gas Bromine vapour ([latex]\scriptsize \displaystyle \text{B}{{\text{r}}_{\text{2}}}[/latex]) is produced. [latex]\scriptsize 2\text{B}{{\text{r}}_{{\text{(aq)}}}}\to \text{ Br}{{_{2}^{{2+}}}_{{\text{(aq)}}}}+2{{\text{e}}^{-}}[/latex] |
Cathode (negative) | Silvery liquid metal present at this electrode underneath the remaining molten electrolyte. Lead ([latex]\scriptsize \displaystyle \text{Pb}[/latex]) is produced. [latex]\scriptsize \text{Pb}_{{\text{(aq)}}}^{{{{\text{2}}^{\text{+}}}}}+\text{2}{{\text{e}}^{\text{-}}}\to \text{P}{{\text{b}}_{{\text{(aq)}}}}[/latex] |
It is easy to predict the products of electrolysis of molten electrolytes because they simply split into their elements:
- the metal is formed at the cathode (negative electrode) because that is where the positive metal ions are attracted.
- the non-metal element is formed at the anode (positive electrode) where the negative non-metal ions are attracted.
Compound | Anode | Cathode |
Zinc Chloride | Chlorine | Zinc |
Aluminium Oxide | Oxygen | Aluminium |
Note
Spectator ions may be either cations (positively charged ions) or anions (negatively charged ions). The ion is unchanged on both sides of a chemical equation and does not affect equilibrium. When writing a net ionic equation, spectator ions found in the original equation are ignored.
Chloralkali industry
Sodium chloride (NaCl), commonly known as salt, is used to treat icy roads and to season food, as well as to produce chemicals for a range of uses. Hydrogen is one of the three products, as well as sodium and chlorine from the electrolysis of sodium chloride solution.
Sodium chloride can be made in a laboratory by reacting sodium with chlorine. However, it is found naturally in large amounts in seawater or in underground deposits. It is often obtained either by evaporating seawater or by mining underground deposits.
Salt is mined by solution mining. Water is pumped down into the salt deposit. Salt dissolves in the water, forming a concentrated salt solution. This is then pumped up to the surface ready for use in the chemical industry. Solution mining is a continuous process that is safer than sending miners underground.
Salt is widely used in the food industry as a preservative and flavour enhancer. It is vital for human health – we need sodium in our diet to allow our bodies to carry out essential functions. Sodium chloride is the raw material for the manufacture of hydrogen, chlorine, and sodium hydroxide using electrolysis. The electrolysis of sodium chloride solution (brine) is an important industrial process because hydrogen, chlorine and sodium hydroxide have many uses.
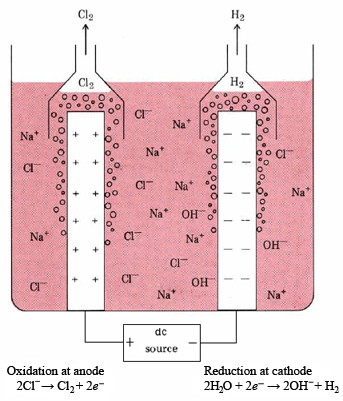
You might have expected sodium metal to be deposited at the negative electrode. However, sodium ions are and do not react. The water in the solution reacts to produce hydrogen. and hydroxide ions.
Since chloride ions are removed and hydroxide ions are produced by the electrolysis, the electrolyte gradually changes from a solution of sodium chloride to a solution of sodium hydroxide.
During electrolysis:
Hydrogen ions [latex]\scriptsize \displaystyle \text{H}_{{\left( {\text{aq}} \right)}}^{\text{+}}[/latex] (from the water) are discharged at the cathode as hydrogen gas, [latex]\scriptsize \displaystyle {{\text{H}}_{{\text{2}\left( \text{g} \right)}}}[/latex]: [latex]\scriptsize \displaystyle \text{2}{{\text{H}}_{\text{2}}}{{\text{O}}_{{\text{(l)}}}}\text{+2}{{\text{e}}^{\text{-}}}\to {{\text{H}}_{{\text{2(g)}}}}\text{+2O}{{\text{H}}^{\text{-}}}_{{\text{(aq)}}}[/latex]
Chloride ions [latex]\scriptsize \displaystyle \text{Cl}_{{\left( {\text{aq}} \right)}}^{\text{--}}[/latex] are discharged at the anode as chlorine gas, [latex]\scriptsize \displaystyle \text{C}{{\text{l}}_{{\text{2}\left( \text{g} \right)}}}[/latex]: [latex]\scriptsize \displaystyle \text{2Cl}_{{_{{\text{(aq)}}}}}^{{^{\text{-}}}}\to \text{C}{{\text{l}}_{\text{2}}}_{{\text{(g)}}}\text{+2}{{\text{e}}^{\text{-}}}[/latex]
Sodium ions [latex]\scriptsize \displaystyle \text{Na}_{{\left( {\text{aq}} \right)}}^{\text{+}}[/latex] and hydroxide ions [latex]\scriptsize \displaystyle \text{OH}_{{\left( {\text{aq}} \right)}}^{\text{--}}[/latex] (from the water) stay behind – they form sodium hydroxide solution, [latex]\scriptsize \displaystyle \text{NaO}{{\text{H}}_{{\left( {\text{aq}} \right)}}}[/latex]
The overall reaction is: [latex]\scriptsize \displaystyle \text{2}{{\text{H}}_{\text{2}}}{{\text{O}}_{{\left( \text{l} \right)}}}\text{+2Cl}_{{\left( {\text{aq}} \right)}}^{\text{-}}\to {{\text{H}}_{{\text{2}\left( \text{g} \right)}}}\text{+C}{{\text{l}}_{{\text{2}\left( \text{g} \right)}}}\text{+2OH}_{{\left( {\text{aq}} \right)}}^{\text{-}}[/latex]
The three products of the electrolysis of concentrated sodium chloride solution have important uses in the chemical industry:
- hydrogen is used as a fuel and for making ammonia
- chlorine is used to sterilise water supplies, and to make bleach and hydrochloric acid
- sodium hydroxide is used to make soap, paper, and bleach.
Electrolytic cells
In an electrical potential energy is converted to chemical potential energy. An electrolytic cell uses an electric current to force a particular chemical reaction to occur, which would otherwise not take place.
Sometimes galvanic cells are called electrochemical cells. While they are electrochemical cells, electrolytic cells are also electrochemical cells. Electrolytic and galvanic cells are not the same, however.
An electrolytic cell is activated by applying an electrical potential across the electrodes to force an internal chemical reaction between the electrodes and the ions that are in the electrolyte solution. This process is called electrolysis.
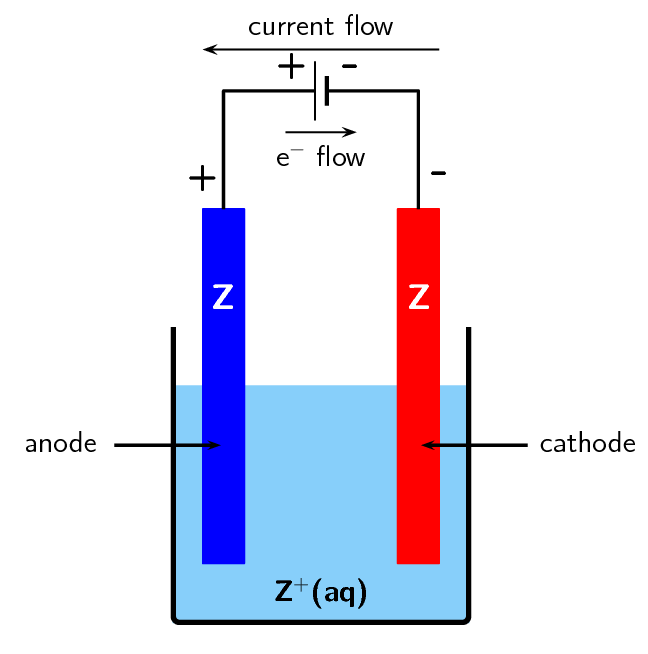
In an electrolytic cell:
- the electrolyte solution consists of the metal cations and spectator anions
- the oxidation and reduction reactions occur in the same container but are non-spontaneous; they require the electrodes to be connected to an external power source to proceed
- the electrodes in an electrolytic cell can be the same metal or different metals; the principle is the same: let there be only one metal, and let that metal be Z
- an electrode is connected to the positive terminal of the battery; oxidation is loss at the anode
- to balance the charge at the anode metal atoms are oxidised to form metal ions; the ions move into solution, leaving their electrons on the electrode
- the following reaction takes place: [latex]\scriptsize \displaystyle {{\text{Z}}_{{\left( \text{s} \right)}}}\to \text{Z}_{{\left( {\text{aq}} \right)}}^{\text{+}}\text{+}{{\text{e}}^{\text{-}}}[/latex]
- an electrode is connected to the negative terminal of the battery; reduction is gain at the cathode
- when positive ions come in contact with the cathode, the ions gain electrons and are reduced
- the following reaction takes place: [latex]\scriptsize \displaystyle \text{Z}_{{\left( {\text{aq}} \right)}}^{\text{+}}\text{+}{{\text{e}}^{\text{-}}}\to {{\text{Z}}_{{\left( \text{s} \right)}}}[/latex]
- this means that the overall reaction is: [latex]\scriptsize \displaystyle {{\text{Z}}_{{\left( \text{s} \right)}}}\text{+ Z}_{{\left( {\text{aq}} \right)}}^{\text{+}}\to \text{Z}_{{\left( {\text{aq}} \right)}}^{\text{+}}\text{+ }{{\text{Z}}_{{\left( \text{s} \right)}}}[/latex].
While this might seem trivial, this is an important technique to purify metals. Water can undergo electrolysis to form hydrogen gas and oxygen gas according to the following reaction:[latex]\scriptsize \displaystyle \text{2}{{\text{H}}_{2}}{{\text{O}}_{{\left( \text{l} \right)}}}\to \text{2}{{\text{H}}_{{\text{2}\left( \text{g} \right)}}}\text{+}{{\text{O}}_{{\text{2}\left( \text{g} \right)}}}[/latex].
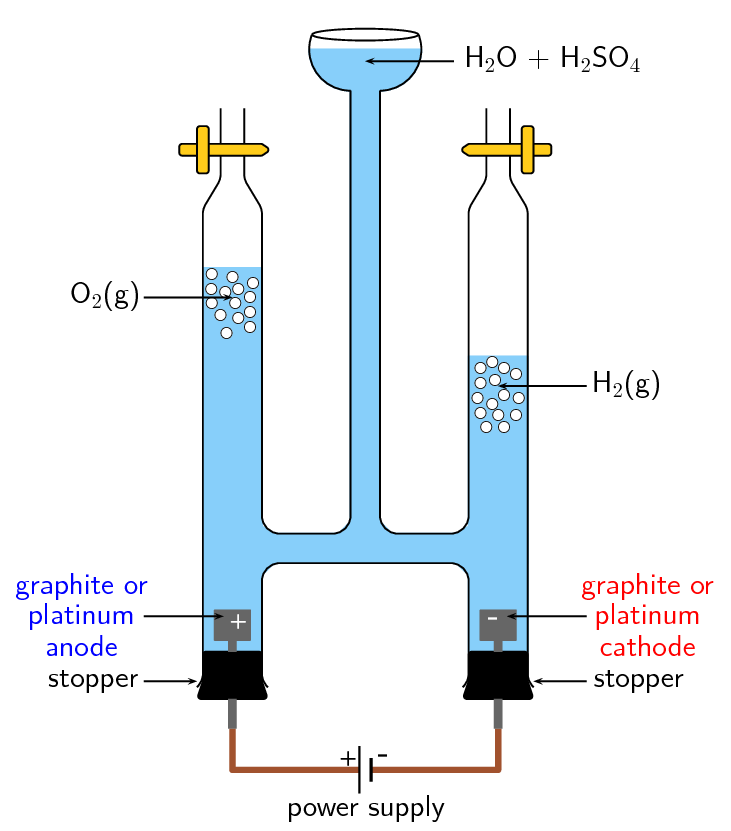
This reaction is very important because hydrogen gas has the potential to be used as an energy source. The electrolytic cell for this reaction consists of two electrodes, submerged in an electrolyte, and connected to a source of electric current.
The oxidation half-reaction is as follows: [latex]\scriptsize \displaystyle \text{4O}{{\text{H}}^{\text{--}}}_{{\left( {\text{aq}} \right)}}\to \text{2}{{\text{H}}_{\text{2}}}{{\text{O}}_{{\left( \text{l} \right)}}}\text{ + }{{\text{O}}_{\text{2}}}_{{\left( \text{g} \right)}}\text{ + 4}{{\text{e}}^{\text{--}}}[/latex]
The reduction half-reaction is as follows: [latex]\scriptsize \displaystyle \text{4}{{\text{H}}^{\text{+}}}_{{\left( {\text{aq}} \right)}}\text{ + 4}{{\text{e}}^{\text{--}}}\to \text{2}{{\text{H}}_{\text{2}}}_{{\left( \text{g} \right)}}[/latex]
Galvanic cells
A (which is also sometimes referred to as a voltaic or wet cell) consists of two half-cells, which convert chemical potential energy into electrical potential energy. In a galvanic cell there are two half-cells. Each contains an electrode in an electrolyte. The separation is necessary to prevent direct chemical contact of the oxidation and reduction reactions, creating a potential difference. The electrons released in the oxidation reaction travel through an external circuit (and do work) before being used by the reduction reaction.
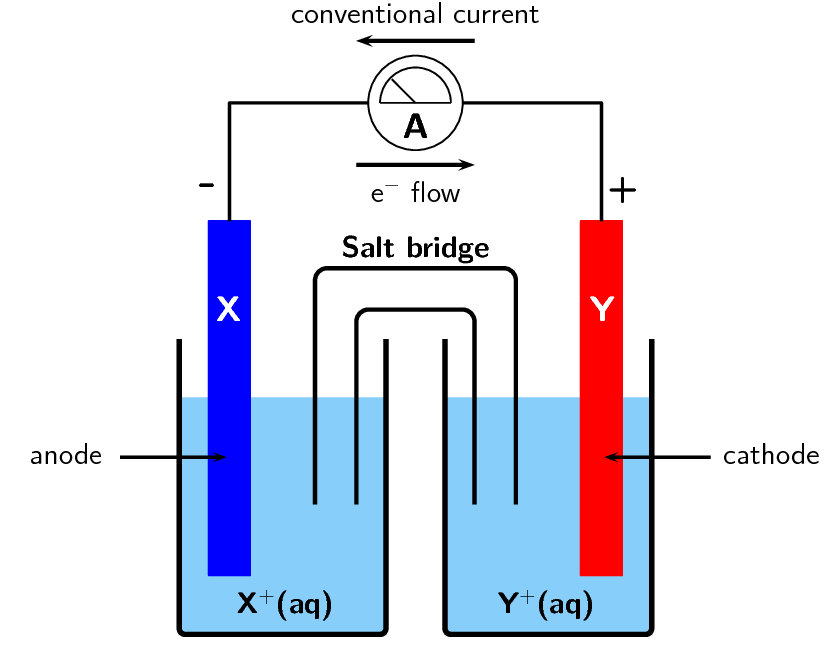
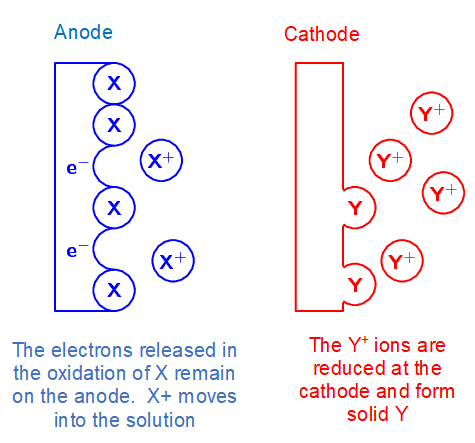
The metal at the anode is X. Oxidation is loss of electrons at the anode.
The anode half-reaction is: [latex]\scriptsize \displaystyle {{\text{X}}_{{\left( \text{s} \right)}}}\to \text{X}_{{\left( {\text{aq}} \right)}}^{\text{+}}\text{ + }{{\text{e}}^{\text{-}}}[/latex]
This half-reaction occurs in the half-cell containing the [latex]\scriptsize \displaystyle {{\text{X}}_{{\left( \text{s} \right)}}}[/latex] anode and the [latex]\scriptsize \displaystyle \text{X}_{{\left( {\text{aq}} \right)}}^{\text{+}}[/latex] electrolyte solution. The electrons released in the oxidation of the metal remain on the anode, while the metal cations formed move into solution.
The metal at the cathode is Y. Reduction is gain of electrons at the cathode.
The cathode half-reaction is: [latex]\scriptsize \displaystyle \text{Y}_{{\left( {\text{aq}} \right)}}^{\text{+}}\text{ + }{{\text{e}}^{\text{-}}}\to {{\text{Y}}_{{\left( \text{s} \right)}}}[/latex]
This half-reaction occurs in the half-cell containing the [latex]\scriptsize \displaystyle {{\text{Y}}_{{\left( \text{s} \right)}}}[/latex] cathode and the [latex]\scriptsize \displaystyle \text{Y}_{{\left( {\text{aq}} \right)}}^{\text{+}}[/latex] electrolyte solution. At the cathode metal ions in the solution are being reduced (accepting electrons) and deposited on the electrode.
Electrons will flow from areas of high concentration to areas of low concentration, therefore the electrons move from the anode, through the external circuit, to the cathode. Conventional current is measured as a flow of positive charge and so is in the opposite direction (from the cathode to the anode).
The overall reaction is: [latex]\scriptsize \displaystyle {{\text{X}}_{{\left( \text{s} \right)}}}\text{+Y}_{{\left( {\text{aq}} \right)}}^{\text{+}}\to \text{X}_{{\left( {\text{aq}} \right)}}^{\text{+}}\text{+}{{\text{Y}}_{{\left( \text{s} \right)}}}[/latex]
To represent this reaction using standard cell notation we write the following: [latex]\scriptsize \displaystyle {{\text{X}}_{{\left( \text{s} \right)}}}\left| {\text{X}_{{\left( {\text{aq}} \right)}}^{\text{+}}} \right|\left| {\text{Y}_{{\left( {\text{aq}} \right)}}^{\text{+}}} \right|{{\text{Y}}_{{\left( \text{s} \right)}}}[/latex]
By convention:
- The anode is always written on the left.
- The cathode is always written on the right.
- The anode and cathode half-cells are divided by || representing the salt bridge.
- The different phases within each half-cell (solid (s) and aqueous (aq) here) are separated by |.
- The electrodes in each half-cell are connected through a wire in the external circuit. There is also a salt bridge between the individual half-cells.
A galvanic cell uses the reactions that take place at the two electrodes to produce electrical energy, i.e. the reaction occurs without the need to add energy.
Note
A galvanic cell generates voltage across their terminals and are a source of energy whilst electrolytic cells need an external potential difference.
Galvanic cell | Electrolytic cell | |
Chemical reactions | spontaneous reactions | non-spontaneous reactions |
Energy changes | Chemical potential energy from chemical reactions is converted to electrical energy | An external supply of electrical energy causes a chemical reaction to occur |
Anode | is negative, oxidation occurs at anode | is positive, oxidation occurs at anode |
Cathode | is positive, reduction occurs at cathode | is negative, reduction occurs at cathode |
Cell set-up | two half-cells, one electrode in each, connected by a salt-bridge | one cell, both electrodes in cell, no salt-bridge |
Electrolyte solution(s) | the electrolyte solutions are kept separate from one another, and are connected by a salt bridge | The cathode and anode are in the same electrolyte |
Applications | batteries | electrolysis e.g. of water, NaCl, electroplating |
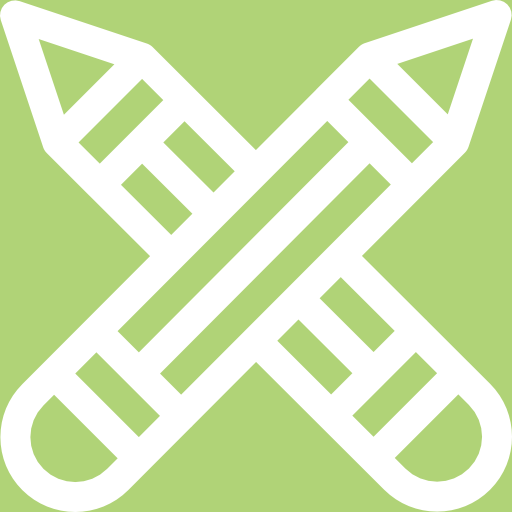
Exercise 1.3
- A galvanic cell consists of two half-cells: a copper anode in a copper nitrate [latex]\scriptsize \displaystyle \left( {\text{Cu}{{{\left( {\text{N}{{\text{O}}_{\text{3}}}} \right)}}_{{\text{2}\left( {\text{aq}} \right)}}}} \right)[/latex] solution, and a silver cathode in a silver nitrate [latex]\scriptsize \displaystyle \text{(AgN}{{\text{O}}_{{\text{3}\left( {\text{aq}} \right)}}}\text{)}[/latex] solution.
- Give equations for the half-reactions that take place at the anode and cathode.
- Write the overall reaction for this cell.
- Give standard cell notation for this cell.
- Draw a simple diagram of the galvanic cell. On your diagram, show the direction in which current flows.
- An electrolytic cell consists of two electrodes in a silver chloride ([latex]\scriptsize \displaystyle \text{AgCl}[/latex]) solution, connected to a source of current. A current is passed through the solution and [latex]\scriptsize \displaystyle \text{A}{{\text{g}}^{\text{+}}}[/latex] ions are reduced to a silver metal deposit on one of the electrodes.
- What is the name of this process?
- Does reduction occur at the electrode where the deposit formed?
- Give the equation for the reduction half-reaction and for the oxidation half-reaction.
The full solutions are at the end of the unit.
Electrochemical reactions
We use batteries throughout our day-to-day lives. Cell phones use lithium-ion batteries, cars use lead-acid batteries, while silver-oxide batteries are used in watches. Some batteries are rechargeable, while others cannot be recharged and have to be thrown away.
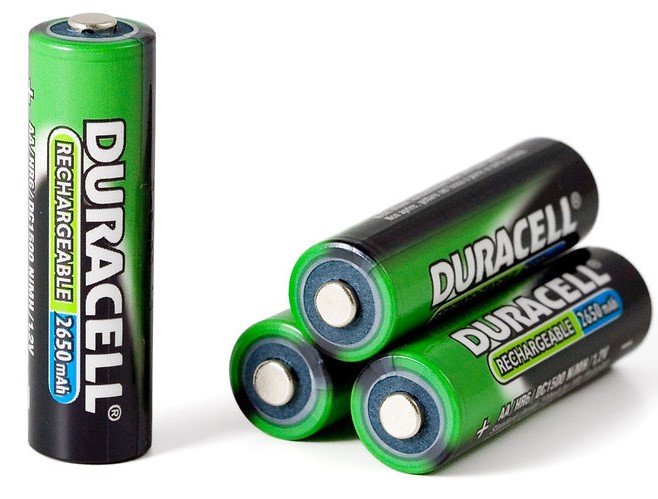
A battery consists of multiple electrochemical cells. And within each cell there are electrochemical reactions taking place. The chemical potential energy can be converted to electrical potential energy, which forms an electric current. The transfer of electrons in a chemical reaction can cause electrical current to flow. If you supply an electric current, it can cause a chemical reaction to take place, by supplying the electrons (and potential energy) necessary for the reactions taking place within the cell.
These types of reactions are called .
An electrochemical reaction is a reaction where either:
- a spontaneous chemical reaction creates an electrical potential difference, and therefore an electric current in the external conducting wires
.
or
. - an electric current provides electrical potential energy and electrons, and therefore forces a non-spontaneous chemical reaction to take place.
Electrochemistry is the branch of chemistry that studies these electrochemical reactions. An is a device in which electrochemical reactions take place.
Electrochemical cells
There are two types of electrodes in an electrochemical cell, the anode, and the cathode. The electrode is an electrical conductor that connects the electrochemical species from its solution to the external electrical circuit of the cell.
Oxidation always occurs at the anode while reduction always occurs at the cathode. So when trying to determine which electrode you are looking at, first determine whether oxidation or reduction is occurring there. An easy way to remember this is:
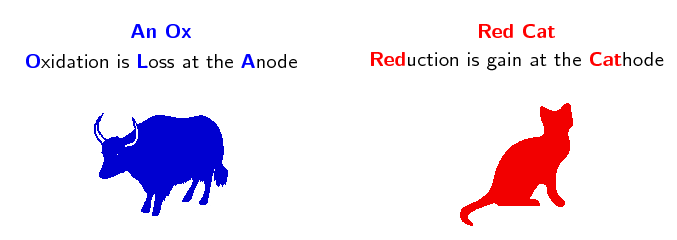
The electrode is placed in an electrolyte solution within the cell. An electrolyte is a solution that contains free ions, and which therefore behaves as a conductor of charges (electrical conductor) in solution.
If the cell is made up of two compartments, as in a galvanic cell, those compartments will be connected by a . A salt bridge is a material which contains electrolytic solution and acts as a connection between two half-cells (and completes the circuit). It maintains electrical neutrality in and between the electrolytes in the half-cell compartments.
The salt bridge acts as a transfer medium that allows ions to flow through without allowing the different solutions to mix and react directly. It allows a balancing of the charges in the electrolyte solutions and allows the reactions in the cell to continue. Without the salt bridge, the flow of electrons in the outer circuit stops completely. This is because the salt bridge is needed to complete the circuit.
Half-cells
Galvanic cells are made up of two half-cells. A half-cell is a structure that consists of a conductive electrode surrounded by a conductive electrolyte. One half-cell contains the anode and an electrolyte containing the same metal cations. The other half-cell contains the cathode and an electrolyte containing the same metal cations. These half-cells are connected by a salt-bridge and the electrodes are connected through an external circuit.
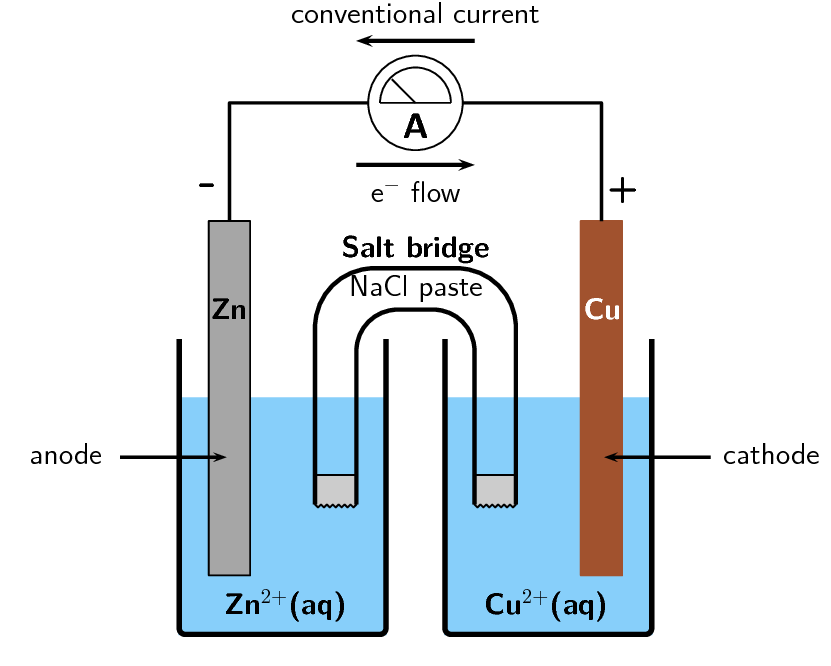
In the diagram of a zinc-copper cell (see figure 12) the cell is made up of a zinc half-cell, containing a zinc electrode and a zinc (II) sulphate ([latex]\scriptsize \displaystyle \text{ZnS}{{\text{O}}_{\text{4}}}[/latex]) electrolyte solution, and a copper half-cell, containing a copper electrode and a copper (II) sulphate solution.
In each half-cell a half-reaction takes place:
At the copper plate, there was an increase in mass. This means that [latex]\scriptsize \displaystyle \text{C}{{\text{u}}^{{\text{2+}}}}[/latex] ions from the copper (II) sulfate solution were deposited onto the plate as atoms of copper metal. The half-reaction that takes place at the copper plate is:
[latex]\scriptsize \displaystyle \text{Cu}_{{\left( {\text{aq}} \right)}}^{{\text{2+}}}\text{+2}{{\text{e}}^{\text{-}}}\to \text{C}{{\text{u}}_{{\left( \text{s} \right)}}}[/latex]
As electrons are gained by the copper ions this is the reduction half-reaction.
At the zinc plate, there was a decrease in mass. This means that some of the solid zinc goes into solution as [latex]\scriptsize \displaystyle \text{Z}{{\text{n}}^{{\text{2+}}}}[/latex] ions. The electrons remain on the zinc plate, giving it a negative charge. The half-reaction that takes place at the zinc plate is:
[latex]\scriptsize \displaystyle \text{Z}{{\text{n}}_{{\left( \text{s} \right)}}}\to \text{Zn}_{{\left( {\text{aq}} \right)}}^{{\text{2+}}}\text{+2}{{\text{e}}^{\text{-}}}[/latex]
As electrons are lost by the zinc atoms this is the oxidation half-reaction.
You can then combine the two half-reactions from these two half-cells to get the overall reaction:
[latex]\scriptsize \displaystyle \text{Z}{{\text{n}}_{{\left( \text{s} \right)}}}\text{+Cu}_{{\left( {\text{aq}} \right)}}^{{\text{2+}}}\text{+2}{{\text{e}}^{\text{-}}}\to \text{Zn}_{{\left( {\text{aq}} \right)}}^{{\text{2+}}}\text{+C}{{\text{u}}_{{\left( \text{s} \right)}}}\text{+2}{{\text{e}}^{\text{-}}}[/latex]
or, if we cancel the electrons:
[latex]\scriptsize \displaystyle \text{Z}{{\text{n}}_{{\left( \text{s} \right)}}}\text{+Cu}_{{\left( {\text{aq}} \right)}}^{{\text{2+}}}\to \text{Zn}_{{\left( {\text{aq}} \right)}}^{{\text{2+}}}\text{+C}{{\text{u}}_{{\left( \text{s} \right)}}}[/latex]
It is possible to look at the half-reaction taking place in a half-cell and determine which electrode is the anode and which is the cathode.
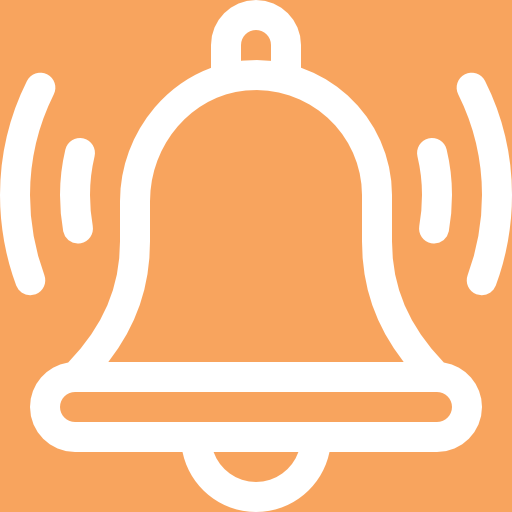
Take note!
Oxidation is loss at the anode; therefore the oxidation half-reaction occurs in the half-cell containing the anode.
Reduction is gain at the cathode, so the reduction half-reaction occurs in the half-cell containing the cathode.
Remember that for an electrochemical cell the standard cell notation is:
[latex]\scriptsize \displaystyle \text{Z}{{\text{n}}_{{\left( \text{s} \right)}}}\left| {\text{Zn}_{{\left( {\text{aq}} \right)}}^{{\text{2+}}}} \right|\left| {\text{Cu}_{{\left( {\text{aq}} \right)}}^{{\text{2+}}}} \right|\text{C}{{\text{u}}_{{\left( \text{s} \right)}}}[/latex]
| = a phase boundary (solid/aqueous)
|| = the salt bridge
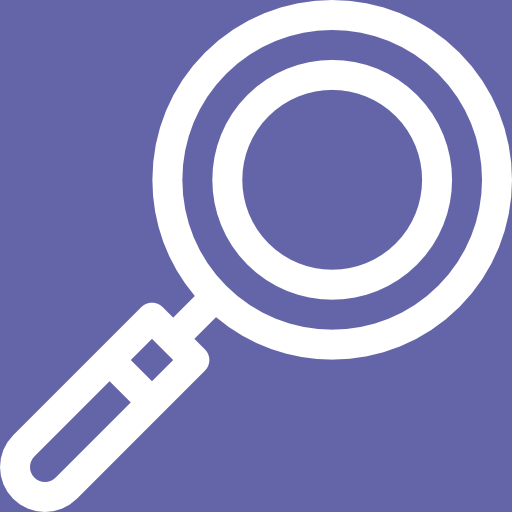
Example 1.2
For the following cell:
[latex]\scriptsize \displaystyle \text{Z}{{\text{n}}_{{\left( \text{s} \right)}}}\left| {\text{Zn}_{{\left( {\text{aq}} \right)}}^{{\text{2+}}}} \right|\left| {\text{Ag}_{{\left( {\text{aq}} \right)}}^{\text{+}}} \right|\text{A}{{\text{g}}_{{\left( \text{s} \right)}}}[/latex]
- Give the anode and cathode half-reactions.
- Write the overall equation for the chemical reaction.
- Give the direction of the current in the external circuit.
- Identify the oxidation and reduction reactions.
Solution
- By convention in standard cell notation, the anode is written on the left and the cathode is written on the right. So, in this cell:
Zinc is the anode (solid zinc is oxidised).
Silver is the cathode (silver ions are reduced). - Write the two half-reactions
Oxidation is loss of electrons at the anode: [latex]\scriptsize \displaystyle \text{Z}{{\text{n}}_{{\left( \text{s} \right)}}}\to \text{Zn}_{{\left( {\text{aq}} \right)}}^{{\text{2+}}}\text{+2}{{\text{e}}^{\text{-}}}[/latex]
Reduction is gain of electrons at the cathode: [latex]\scriptsize \displaystyle \text{Ag}_{{\left( {\text{aq}} \right)}}^{\text{+}}\text{+}{{\text{e}}^{\text{-}}}\to \text{A}{{\text{g}}_{{\left( \text{s} \right)}}}[/latex]
Combine the half-reactions to get the overall equation
Balance the charge by multiplying the reduction half-reaction by [latex]\scriptsize \displaystyle 2[/latex]
[latex]\scriptsize \displaystyle \begin {align*}\text{2Ag}_{{\left( {\text{aq}} \right)}}^{\text{+}}\text{+2}{{\text{e}}^{\text{-}}}&\to \text{2A}{{\text{g}}_{{\left( \text{s} \right)}}} \\ \text{Z}{{\text{n}}_{{\left( \text{s} \right)}}}\text{+2Ag}_{{\left( {\text{aq}} \right)}}^{\text{+}}&\to \text{Zn}_{{\left( {\text{aq}} \right)}}^{{\text{2+}}}\text{+A}{{\text{g}}_{{\left( \text{s} \right)}}} \end{align*}[/latex] - Electron flow is from negative to positive, so from the anode to the cathode. Conventional current is in the opposite direction to electron flow. Therefore current will flow from the cathode (silver) to the anode (zinc).
- The solid zinc is oxidised to form zinc ions. These electrons are left on the zinc electrode (anode) making it negative.
The silver ions take electrons and are reduced to form solid silver. This makes the silver electrode (cathode) positive.
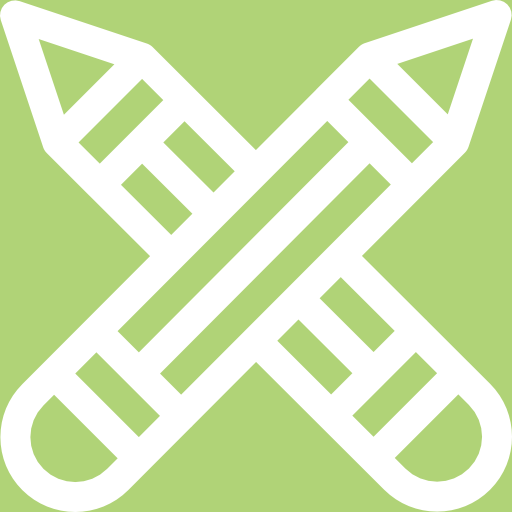
Exercise 1.4
- In each of the following equations, say which elements in the reactants are oxidised and which are reduced.
- [latex]\scriptsize \displaystyle \text{Cu}{{\text{O}}_{{\left( \text{s} \right)}}}\text{+}{{\text{H}}_{{\text{2}\left( \text{g} \right)}}}\to \text{C}{{\text{u}}_{{\left( \text{s} \right)}}}\text{+}{{\text{H}}_{\text{2}}}{{\text{O}}_{{\left( \text{g} \right)}}}[/latex]
- [latex]\scriptsize \displaystyle \text{2N}{{\text{O}}_{{\left( \text{g} \right)}}}\text{+2C}{{\text{O}}_{{\left( \text{g} \right)}}}\to {{\text{N}}_{{\text{2}\left( \text{g} \right)}}}\text{+2C}{{\text{O}}_{{\text{2}\left( \text{g} \right)}}}[/latex]
- [latex]\scriptsize \displaystyle \text{M}{{\text{g}}_{{\left( \text{s} \right)}}}\text{+FeS}{{\text{O}}_{{\text{4}\left( {\text{aq}} \right)}}}\to \text{MgS}{{\text{O}}_{{\text{4}\left( {\text{aq}} \right)}}}\text{+F}{{\text{e}}_{{\left( \text{s} \right)}}}[/latex]
- The following half-reactions take place in an electrochemical cell:
[latex]\scriptsize \displaystyle \begin{align*}\text{F}{{\text{e}}_{{\left( \text{s} \right)}}}&\to \text{Fe}_{{\left( {\text{aq}} \right)}}^{{\text{3+}}}\text{+3}{{\text{e}}^{\text{-}}} \\ \text{Fe}_{{\left( {\text{aq}} \right)}}^{{\text{2+}}}\text{+2}{{\text{e}}^{\text{-}}}&\to \text{F}{{\text{e}}_{{\left( \text{s} \right)}}} \end{align*}[/latex]- Which is the oxidation half-reaction?
- Which is the reduction half-reaction?
- Name the oxidising agent.
- Name the reducing agent.
- Use standard notation to represent this electrochemical cell.
- For the following cell:
[latex]\scriptsize \displaystyle \text{M}{{\text{g}}_{{\left( \text{s} \right)}}}\left| {\text{Mg}_{{\left( {\text{aq}} \right)}}^{{\text{2+}}}} \right|\left| {\text{Mn}_{{\left( {\text{aq}} \right)}}^{{\text{2+}}}} \right|\text{M}{{\text{n}}_{{\left( \text{s} \right)}}}[/latex]- Give the cathode and the anode half-reactions.
- Give the overall equation for the electrochemical cell.
- What metals could be used for the electrodes in this electrochemical cell?
- Suggest two electrolytes for this electrochemical cell.
- Draw a simple sketch of the complete cell.
The full solutions are at the end of the unit.
Note
To understand galvanic cells reactions and half cells watch this video by M Champagne, called Galvanic Cells and Activity Series La.
Battery types
Primary batteries are non-rechargeable batteries. They include zinc carbon batteries, alkaline batteries, button cell batteries and lithium batteries.
- The zinc carbon battery is labelled as ‘all purpose’ or ‘general purpose’ and discharges quickly if used continuously. The zinc chloride cells are longer lasting and are labelled ‘heavy duty’ or ‘super heavy duty’.
- Alkaline batteries can last up to ten times longer than zinc batteries but may cost three to five times more.
- Button cell batteries are small, disc-shaped batteries commonly used in hearing aids, medical devices, watches, calculators, and cameras.
- Lithium batteries can last about twice as long as alkaline batteries but are more expensive. Lithium batteries are labelled as such to distinguish them from other battery types.
Secondary batteries are rechargeable batteries. They are available as freestanding units or as built-in components of rechargeable devices. The free-standing units are expensive but save money in the long run since they can be recharged many times. They are the best for devices that get regular use. The most common types include nickel cadmium (Ni-Cd), sealed lead-acid (Pb), nickel metal hydride (Ni- MH), and lithium ion (Li- Ion).
- Nickel-cadmium is the most common type of rechargeable battery. They may be built into rechargeable appliances or sold as freestanding units. A single nickel cadmium battery can replace about 150 alkaline batteries.
Figure 13: A simplified diagram of a nickel cadmium battery .
A nickel-cadmium cell has two plates. The active material of the anode is [latex]\scriptsize \displaystyle \text{Ni}{{\left( {\text{OH}} \right)}_{\text{4}}}[/latex] and the cathode is of cadmium (Cd) when fully charged. The electrolyte is a solution of potassium hydroxide (KOH) with a small addition of lithium hydrate which increases the capacity and life of the battery. When the cell is fully charged, its positive plate is of Ni(OH)4 and its negative plate is of cadmium (Cd).
.
Discharging: When the cell discharges, the potassium hydroxide (KOH) is dissociated into potassium ([latex]\scriptsize \displaystyle {{\text{K}}^{\text{+}}}[/latex]) and hydroxyl ([latex]\scriptsize \displaystyle \text{O}{{\text{H}}^{\text{--}}}[/latex]) ions. The hydroxyl ions go to the cathode and potassium ions go to the anode. The following chemical reaction takes place during discharging.
-
- At cathode: [latex]\scriptsize \displaystyle \text{C}{{\text{d}}^{+}}\text{ + 2O}{{\text{H}}^{-}}\to \text{Cd}{{\left( {\text{OH}} \right)}_{\text{2}}}+2{{\text{e}}^{-}}[/latex]
- At anode: [latex]\scriptsize \displaystyle \text{Ni}{{\left( {\text{OH}} \right)}_{{\text{4 }}}}\text{+ }{{\text{H}}_{\text{2}}}\text{O + 2}{{\text{e}}^{-}}\to \text{ 2O}{{\text{H}}^{-}}\text{ + Ni}{{\left( {\text{OH}} \right)}_{\text{2}}}[/latex]
The anode is converted from [latex]\scriptsize \displaystyle \text{Ni}{{\left( {\text{OH}} \right)}_{\text{4}}}[/latex] to [latex]\scriptsize \displaystyle \text{NI}{{\left( {\text{OH}} \right)}_{\text{2}}}[/latex] and cathode is converted from cadmium (Cd) to cadmium hydroxide [latex]\scriptsize \displaystyle \text{Cd(OH}{{\text{)}}_{\text{2}}}[/latex].
Charging: When the battery is charged, the hydroxyl ([latex]\scriptsize \displaystyle \text{O}{{\text{H}}^{\text{--}}}[/latex]) ions move towards the anode, whereas the potassium ions ([latex]\scriptsize \displaystyle {{\text{K}}^{\text{+}}}[/latex]) move towards the cathode.
Since the voltage produced by a single cell is very low, many cells are connected in series to get the desired voltage output and this arrangement is known as the nickel-cadmium battery.
- Sealed lead-acid batteries are used in some camcorders and cellular phones. They are less expensive, but much heavier than other types of rechargeable batteries.
Figure 14: A simplified diagram of a lead acid battery .
The reactions in a lead acid battery is:
Cathode: [latex]\scriptsize \displaystyle \text{Pb}{{\text{O}}_{{\text{2}\left( \text{s} \right)}}}\text{+HSO}_{{\left( {\text{aq}} \right)}}^{{\text{-4}}}\text{+3H}_{{\left( {\text{aq}} \right)}}^{\text{+}}\text{+2}{{\text{e}}^{\text{-}}}\to \text{PbS}{{\text{O}}_{{\text{4}\left( \text{s} \right)}}}\text{+2}{{\text{H}}_{\text{2}}}{{\text{O}}_{{\left( \text{l} \right)}}}[/latex]
Anode (oxidation): [latex]\scriptsize \displaystyle \text{P}{{\text{b}}_{{\left( \text{s} \right)}}}\text{+HSO}_{{\left( {\text{aq}} \right)}}^{{\text{-4}}}\to \text{PbS}{{\text{O}}_{{\text{4}\left( \text{s} \right)}}}\text{+H}_{{\left( {\text{aq}} \right)}}^{\text{+}}\text{+2}{{\text{e}}^{\text{-}}}[/latex]
Overall: [latex]\scriptsize \displaystyle \text{P}{{\text{b}}_{{\left( \text{s} \right)}}}\text{+Pb}{{\text{O}}_{{\text{2}\left( \text{s} \right)}}}\text{+2HSO}_{{\left( {\text{aq}} \right)}}^{{\text{4-}}}\text{+2H}_{{\left( {\text{aq}} \right)}}^{\text{+}}\to \text{2PbS}{{\text{O}}_{{\text{4}\left( \text{s} \right)}}}\text{+2}{{\text{H}}_{\text{2}}}{{\text{O}}_{{\left( \text{l} \right)}}}[/latex]
.
The diluted sulfuric acid molecules break into two parts when the acid dissolves. It will create positive ions [latex]\scriptsize \displaystyle \text{2}{{\text{H}}^{\text{+}}}[/latex] and negative ions [latex]\scriptsize \displaystyle \text{S}{{\text{O}}^{{\text{4-}}}}[/latex].
.
Charging: To charge a lead-acid battery we need a charging voltage higher than the nominal battery voltage. To charge a battery of [latex]\scriptsize \displaystyle \text{12 V}[/latex] we need to apply at least [latex]\scriptsize \displaystyle 13.5\text{ V}[/latex] DC to the battery terminals for charging.
Discharging: During the discharge of a battery, lead sulfate crystals form at both the negative and positive terminals, as well as the release of electrons due to the change in valence charge of the lead. This formation of lead sulfate uses sulfate from the sulfuric acid electrolyte surrounding the battery.
.
The charging reaction converts the lead sulfate at the negative electrode to lead. At the positive terminal, the reaction converts the lead to lead oxide. As a by-product of this reaction, hydrogen is evolved. During the first part of the charging cycle, the conversion of lead sulfate to lead and lead oxide is the dominant reaction. As the charging goes on and most of the lead sulfate is converted to either lead or lead dioxide, the charging current electrolyses the water from the electrolyte and both hydrogen and oxygen gas are evolved, a process known as the ‘gassing’ of the battery. - Nickel metal hydride batteries are used in computers, cellular phones, and camcorders. They have a low drain and high-energy capacity.
- Lithium-ion batteries are very expensive, but extremely light and high in energy density. They are used in some cellular phones and notebook computers.
.
In these batteries ions of lithium travel from negative electrodes to positive electrodes inside the battery through the electrolyte during discharge or when a load is connected, and vice versa (positive to the negative electrode ) during charging.
These are very high-quality batteries that have high power density and a very low self-discharge which is very good quality.Figure 15: The basic structure of lithium-ion batteries
Sacrificial anodes and the protection of metals
is a metal coating process in which a ferrous part is coated with a thin layer of zinc. The zinc coating seals the surface of the part from the environment, preventing oxidation and weathering. Galvanisation helps to extend the life of steel parts by providing a barrier between the steel and the atmosphere, preventing iron oxide from forming on the surface of the steel. Galvanisation also provides superior corrosion resistance to parts exposed to the environment. Galvanisation provides a cost-effective solution for coating steel parts, specifically those that will receive significant environmental exposure over their lifetime.
The primary method of galvanisation is ‘hot dip galvanization’, which has been in use for over 150 years. Sacrificial anodes are highly active metals that are used to prevent a less active material surface from corroding. Sacrificial anodes are created from a metal alloy with a more negative electrochemical potential than the other metal it will be used to protect. The sacrificial anode will be consumed in place of the metal it is protecting, which is why it is referred to as a ‘sacrificial’ anode.
When metal surfaces come into contact with electrolytes, they undergo an electrochemical reaction known as corrosion. Corrosion is the process of returning a metal to its natural state as an ore and in this process, causing the metal to disintegrate and its structure to grow weak. These metal surfaces are used all around us — from pipelines to buildings to ships. It is important to ensure that these metals last as long as they can and thus necessitates what is known as cathode protection.
Sacrificial anodes are among several forms of cathode protection. Other forms of cathode protection are plating, galvanisation, and the formation of alloys.
Metal in seawater is one such example with the iron metal coming into contact with electrolytes. Under normal circumstances, the iron metal would react with the electrolytes and begin to corrode, growing weaker in structure and disintegrating. The addition of zinc, a sacrificial anode, would prevent the iron metal from ‘corroding’. According to the Table of Standard Reduction Potentials, the standard reduction potential of zinc is about [latex]\scriptsize \displaystyle -0.76\text{ v}[/latex]. The standard reduction potential of iron is about [latex]\scriptsize \displaystyle -0.44\text{ v}[/latex]. This difference in reduction potential means that zinc would oxidise much faster than iron would. In fact, zinc would oxidise completely before iron would begin to react.
The materials used for sacrificial anodes are either relatively pure active metals, such as zinc or magnesium, or are magnesium or aluminium alloys that have been specifically developed for use as sacrificial anodes. In applications where the anodes are buried, a special backfill material surrounds the anode to ensure that the anode will produce the desired output.
Since the sacrificial anode works by introducing another metal surface with a more negative electronegative and much more anodic surface. The current will flow from the newly introduced anode and the protected metal becomes cathodic creating a galvanic cell. The oxidation reactions are transferred from the metal surface to the galvanic anode and will be sacrificed in favour of the protected metal structure.
Figure 16: A partially corroded sacrificial anode on the hull of a shipSacrificial anodes are normally supplied with lead wires to facilitate their connection to the structure being protected. The lead wires may be attached to the structure by welding or mechanical connections. These should have a low resistance and should be insulated to prevent increased resistance or damage due to corrosion. When anodes with cast-in straps are used, the straps can either be welded directly to the structure or the straps can be used as locations for attachment.
A low resistance mechanically adequate attachment is required for good protection and resistance to mechanical damage. In the process of providing electrons for the cathodic protection of a less active metal the more active metal corrodes. The more active metal (anode) is sacrificed to protect the less active metal (cathode). The amount of corrosion depends on the metal being used as an anode but is directly proportional to the amount of current supplied.
Sacrificial anodes are used to protect the hulls of ships, water heaters, pipelines, distribution systems, above-ground tanks, underground tanks, and refineries. The anodes in sacrificial anode cathodic protection systems must be periodically inspected and replaced when consumed.
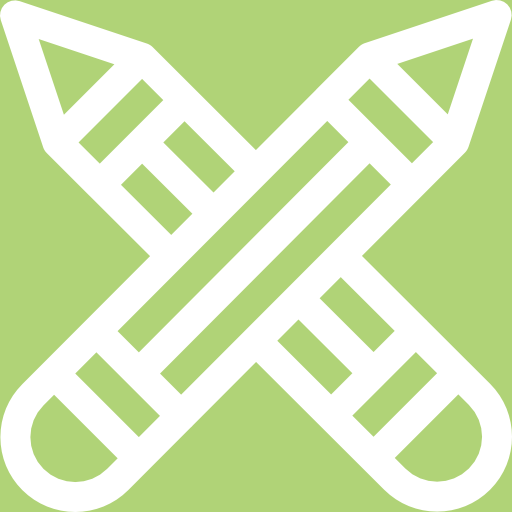
Exercise 1.5
- What are the purposes of using sacrificial anodes?
- How do sacrificial anode’s function?
- What are other forms of cathode protection?
The full solutions are at the end of the unit.
Summary
In this unit you have learnt the following:
- Oxidation is the loss of electrons and reduction is the gain of electrons.
- A redox reaction is one where there is always a change in the oxidation numbers (charge) of the elements that are involved in the reaction.
- It is possible to balance redox equations using the half-reactions that take place within the overall reaction.
- An electrochemical reaction is one where either a chemical reaction produces an electric current, or where an electric current causes a chemical reaction to take place.
- In a galvanic cell a chemical reaction produces a current in the external circuit. An example is the zinc-copper cell.
- An electrolytic cell is an electrochemical cell that uses electricity to drive a non-spontaneous reaction. In an electrolytic cell, electrolysis occurs, which is a process of separating elements and compounds using an electric current.
- Cells have a number of components. They consist of two electrodes, which are connected to each other by an external circuit wire.
- In a galvanic cell each electrode is placed in a separate container in an electrolyte solution. The two electrolytes are connected by a salt bridge.
- In an electrolytic cell both electrodes are placed in the same container in an electrolyte solution.
- One of the electrodes is the anode, where oxidation takes place. The cathode is the electrode where reduction takes place.
- In a galvanic cell, the build-up of electrons at the anode sets up a potential difference between the two electrodes, and this causes a current to flow in the external circuit.
- Standard cell notation for a galvanic cell has the anode on the left and the cathode on the right. For example:
[latex]\scriptsize \displaystyle \text{Z}{{\text{n}}_{{\left( \text{s} \right)}}}\left| {\text{Zn}_{{\left( {\text{aq}} \right)}}^{{\text{2+}}}} \right|\left| {\text{Cu}_{{\left( {\text{aq}} \right)}}^{{\text{2+}}}} \right|\text{C}{{\text{u}}_{{\left( \text{s} \right)}}}[/latex]
| = a phase boundary (solid/aqueous)
|| = the salt bridge - There are different types of batteries: disposable (single use) batteries and rechargeable batteries.
- Sacrificial anodes are more reactive metals used to protect less reactive metals.
Unit 1: Assessment
Suggested time to complete: 30 minutes
- Permanganate (VII) ions ([latex]\scriptsize \displaystyle \text{Mn}{{\text{O}}^{{\text{-4}}}}[/latex]) oxidise hydrogen peroxide ([latex]\scriptsize \displaystyle {{\text{H}}_{\text{2}}}{{\text{O}}_{\text{2}}}[/latex]) to oxygen gas. The reaction is done in an acid medium. During the reaction, the permanganate (VII) ions are reduced to manganese (II) ions ([latex]\scriptsize \displaystyle \text{M}{{\text{n}}^{{\text{2+}}}}[/latex]). Write a balanced equation for the reaction.
- Which one of the substances listed below acts as the oxidising agent in the following reaction?
[latex]\scriptsize \displaystyle \text{3S}{{\text{O}}_{{\text{2}\left( \text{g} \right)}}}\text{+C}{{\text{r}}_{\text{2}}}\text{O}_{{\text{2}\left( {\text{aq}} \right)}}^{{\text{-7}}}\text{+2H}_{{\left( {\text{aq}} \right)}}^{\text{+}}\to \text{3SO}_{{\text{2}\left( {\text{aq}} \right)}}^{{\text{-4}}}\text{+2Cr}_{{\left( {\text{aq}} \right)}}^{{\text{3+}}}\text{+}{{\text{H}}_{\text{2}}}{{\text{O}}_{{\left( \text{l} \right)}}}[/latex]- [latex]\scriptsize \displaystyle {{\text{H}}^{\text{+}}}[/latex]
- [latex]\scriptsize \displaystyle \text{C}{{\text{r}}^{{\text{3+}}}}[/latex]
- [latex]\scriptsize \displaystyle \text{S}{{\text{O}}_{\text{2}}}[/latex]
- [latex]\scriptsize \displaystyle \text{C}{{\text{r}}_{\text{2}}}{{\text{O}}_{\text{2}}}^{{\text{-7}}}[/latex]
- For the following cell:
[latex]\scriptsize \displaystyle \text{S}{{\text{n}}_{{\left( \text{s} \right)}}}\left| {\text{Sn}_{{\left( {\text{aq}} \right)}}^{{\text{2+}}}} \right|\left| {\text{Ag}_{{\left( {\text{aq}} \right)}}^{\text{+}}} \right|\text{A}{{\text{g}}_{{\left( \text{s} \right)}}}[/latex]- Give the cathode and anode half-reactions.
- Give the overall equation for the electrochemical cell.
- Draw a simple sketch of the complete cell.
- Look at the following half-reactions:
[latex]\scriptsize \displaystyle \begin{align*}\text{Ca}_{{\left( {\text{aq}} \right)}}^{{\text{2+}}}\text{+2}{{\text{e}}^{\text{-}}}&\to \text{C}{{\text{a}}_{{\left( \text{s} \right)}}} \\ \text{C}{{\text{l}}_{{\text{2}\left( \text{g} \right)}}}\text{+2}{{\text{e}}^{\text{-}}}&\to \text{2Cl}_{{\left( {\text{aq}} \right)}}^{\text{-}} \\ \text{Fe}_{{\left( {\text{aq}} \right)}}^{{\text{3+}}}\text{+3}{{\text{e}}^{\text{-}}}&\to \text{F}{{\text{e}}_{{\left( \text{s} \right)}}} \\ {{\text{I}}_{{\text{2}\left( \text{s} \right)}}}\text{+2}{{\text{e}}^{\text{-}}}&\to \text{2I}_{{\left( {\text{aq}} \right)}}^{\text{-}} \end{align*}[/latex]- Which substance is the strongest oxidising agent?
- Which substance is the strongest reducing agent?
- A cell is set up. There is a negative charge on the anode.
- What type of cell is this?
- What type of reaction is occurring at the cathode?
- Can the electrodes have the same reaction potential?
- For each of the following, say whether the statement is true or false. If it is false, re-write the statement correctly.
- The anode in an electrolytic cell has a negative charge.
- The reaction [latex]\scriptsize \displaystyle \text{2KCl}{{\text{O}}_{{\text{3}\left( \text{s} \right)}}}\to \text{2KC}{{\text{l}}_{{\left( \text{s} \right)}}}\text{+3}{{\text{O}}_{{\text{2}\left( \text{g} \right)}}}[/latex] is an example of a redox reaction.
- Lead is a stronger oxidising agent than nickel.
- For each of the following questions, choose the one correct answer.
- Which one of the following reactions is a redox reaction?
- [latex]\scriptsize \text{HC}{{\text{l}}_{{\left( {\text{aq}} \right)}}}\text{+NaO}{{\text{H}}_{{\left( {\text{aq}} \right)}}}\to \text{NaC}{{\text{l}}_{{\left( {\text{aq}} \right)}}}\text{+}{{\text{H}}_{\text{2}}}{{\text{O}}_{{\left( \text{l} \right)}}}[/latex]
- [latex]\scriptsize \text{AgN}{{\text{O}}_{{\text{3}\left( \text{s} \right)}}}\text{+Na}{{\text{I}}_{{\left( \text{s} \right)}}}\to \text{Ag}{{\text{I}}_{{\left( \text{s} \right)}}}\text{+NaN}{{\text{O}}_{{\text{3}\left( \text{s} \right)}}}[/latex]
- [latex]\scriptsize \text{2FeC}{{\text{l}}_{{\text{3}\left( {\text{aq}} \right)}}}\text{+2}{{\text{H}}_{\text{2}}}{{\text{O}}_{{\left( \text{l} \right)}}}\text{+S}{{\text{O}}_{{\text{2}\left( {\text{aq}} \right)}}}\to {{\text{H}}_{\text{2}}}\text{S}{{\text{O}}_{{\text{4}\left( {\text{aq}} \right)}}}\text{+2HC}{{\text{l}}_{{\left( {\text{aq}} \right)}}}\text{+2FeC}{{\text{l}}_{{\text{2}\left( {\text{aq}} \right)}}}[/latex]
- [latex]\scriptsize \text{BaC}{{\text{l}}_{{\text{2}\left( \text{s} \right)}}}\text{+MgS}{{\text{O}}_{{\text{4}\left( \text{s} \right)}}}\to \text{MgC}{{\text{l}}_{{\text{2}\left( \text{s} \right)}}}\text{+BaS}{{\text{O}}_{{\text{4(s)}}}}[/latex]
- Consider the reaction represented by the following equation:
[latex]\scriptsize \displaystyle \text{B}{{\text{r}}_{{\text{2}\left( \text{l} \right)}}}\text{+2I}_{{\left( {\text{aq}} \right)}}^{\text{-}}\to \text{2Br}_{{\left( {\text{aq}} \right)}}^{\text{-}}\text{+}{{\text{I}}_{{\text{2}\left( \text{s} \right)}}}[/latex]
Which one of the following statements about this reaction is correct?- bromine is oxidised.
- bromine acts as a reducing agent.
- the iodide ions are oxidised.
- iodine acts as a reducing agent.
- The following equations represent two hypothetical half-reactions:
[latex]\scriptsize \displaystyle {{\text{X}}_{\text{2}}}\text{+2}{{\text{e}}^{\text{-}}}\rightleftharpoons \text{2}{{\text{X}}^{\text{-}}}\left( {{{\text{E}}^{\text{o}}}\text{= +1}\text{.09 V}} \right)[/latex]
and
[latex]\scriptsize \displaystyle {{\text{Y}}^{\text{+}}}\text{+}{{\text{e}}^{\text{-}}}\rightleftharpoons \text{Y }\left( {{{\text{E}}^{\text{o}}}\text{=-2}\text{.80 V}} \right)[/latex]
Which one of the following substances from these half-reactions has the greatest tendency to lose electrons?- [latex]\scriptsize {\text{X}}^{\text{-}}[/latex]
- [latex]\scriptsize {\text{X}}_{\text{2}}[/latex]
- [latex]\scriptsize \text{Y}[/latex]
- [latex]\scriptsize {\text{Y}}^{\text{+}}[/latex]
- Which one of the following reactions is a redox reaction?
The full solutions are at the end of the unit.
Unit 1: Solutions
Exercise 1.1
- .
- Oxidation is the loss of electrons by a molecule, atom, or ion.
- Reduction is the gain of electrons by a molecule, atom, or ion.
- The molecule, atom or ion that is reduced, or the molecule, atom or ion that causes oxidation.
- The molecule, atom or ion that is oxidised, or the molecule, atom or ion that causes reduction.
- .
- The oxidation number of [latex]\scriptsize \displaystyle \text{Fe}[/latex] in:
[latex]\scriptsize \displaystyle \begin{align*}&\text{F}{{\text{e}}_{{\left( \text{s} \right)}}}\text{ is 0} \\& \text{F}{{\text{e}}^{{\text{2+}}}}_{{\left( {\text{aq}} \right)}}\text{ is +2} \\& \text{F}{{\text{e}}_{{\left( \text{s} \right)}}}\to \text{F}{{\text{e}}^{{\text{2+}}}}_{{\left( {\text{aq}} \right)}}\text{+2}{{\text{e}}^{\text{-}}} \end{align*}[/latex]
[latex]\scriptsize \displaystyle \text{Fe}[/latex] loses two electrons to become [latex]\scriptsize \displaystyle \text{F}{{\text{e}}^{{\text{2+}}}}[/latex]. The iron species ([latex]\scriptsize \displaystyle \text{F}{{\text{e}}_{{\left( \text{s} \right)}}}[/latex]) is oxidised. - The oxidation number of [latex]\scriptsize \displaystyle \text{O}[/latex] is [latex]\scriptsize \displaystyle -2[/latex]. Therefore the oxidation number of [latex]\scriptsize \displaystyle {{\text{O}}_{\text{3}}}[/latex] in [latex]\scriptsize \displaystyle \text{F}{{\text{e}}_{\text{2}}}{{\text{O}}_{\text{3}}}[/latex] is [latex]\scriptsize \displaystyle -6[/latex] and the oxidation number of [latex]\scriptsize \displaystyle \text{F}{{\text{e}}_{\text{2}}}[/latex] in [latex]\scriptsize \displaystyle \text{F}{{\text{e}}_{\text{2}}}{{\text{O}}_{\text{3}}}[/latex] is [latex]\scriptsize \displaystyle +6[/latex].
The oxidation number of [latex]\scriptsize \displaystyle \text{Fe}[/latex] in:
[latex]\scriptsize \displaystyle \begin{align*}&\text{F}{{\text{e}}_{\text{2}}}{{\text{O}}_{{\text{3}\left( \text{s} \right)}}}\text{ is }+3 \\& \text{F}{{\text{e}}_{{\left( \text{s} \right)}}}\text{ is }0 \\& \text{F}{{\text{e}}^{{\text{3+}}}}_{{\left( \text{s} \right)}}\text{+3}{{\text{e}}^{\text{-}}}\to \text{F}{{\text{e}}_{{\left( \text{s} \right)}}} \end{align*}[/latex]
[latex]\scriptsize \displaystyle \text{F}{{\text{e}}^{{\text{3+}}}}[/latex] gains three electrons to become [latex]\scriptsize \displaystyle \text{F}{{\text{e}}_{{\left( \text{s} \right)}}}\text{.}[/latex] The iron species ([latex]\scriptsize \displaystyle \text{F}{{\text{e}}^{{\text{3+}}}}_{{\left( \text{s} \right)}}[/latex]) is reduced.
- The oxidation number of [latex]\scriptsize \displaystyle \text{Fe}[/latex] in:
Exercise 1.2
- .
- [latex]\scriptsize \displaystyle \text{14}{{\text{H}}^{\text{+}}}\text{+2M}{{\text{n}}^{{\text{2+ }}}}\text{+ 5BiO}_{\text{3}}^{\text{-}}\to \text{2MnO}_{\text{4}}^{\text{-}}\text{+ 5Bi}_{\text{3}}^{\text{+}}\text{+7}{{\text{H}}_{\text{2}}}\text{O }[/latex]
- [latex]\scriptsize \displaystyle \text{8}{{\text{H}}_{\text{2}}}\text{O + 2P + 5C}{{\text{u}}^{{\text{2+}}}}\to \text{2}{{\text{H}}_{\text{2}}}\text{PO}_{4}^{-}\text{ + 12}{{\text{H}}^{\text{+}}}\text{+ 5Cu}[/latex]
- [latex]\scriptsize \displaystyle {{\text{H}}_{\text{2}}}\text{O + 3N}{{\text{O}}_{{\text{2 }}}}\to \text{2NO}_{\text{3}}^{\text{-}}\text{+ NO + 2}{{\text{H}}^{\text{+}}}\text{ }[/latex]
- .
- [latex]\scriptsize \displaystyle \text{2Al + 2}{{\text{H}}_{\text{2}}}\text{O + 2O}{{\text{H}}^{\text{-}}}\to \text{2AlO}_{2}^{-}\text{+ 3}{{\text{H}}_{\text{2}}}[/latex]
- [latex]\scriptsize \displaystyle \text{8O}{{\text{H}}^{{\text{- }}}}\text{+ 5P}{{\text{b}}^{{\text{2+}}}}\text{+ 2IO}_{3}^{-}\to \text{5Pb}{{\text{O}}_{\text{2}}}\text{ + }{{\text{I}}_{\text{2}}}\text{ + 4}{{\text{H}}_{\text{2}}}\text{O}[/latex]
Exercise 1.3
- .
- The copper electrode is the anode, oxidation is a loss of electrons at the anode:
Anode half-reaction: [latex]\scriptsize \displaystyle \text{C}{{\text{u}}_{{\left( \text{s} \right)}}}\to \text{Cu}_{{\left( {\text{aq}} \right)}}^{{\text{2+}}}\text{+2}{{\text{e}}^{\text{-}}}[/latex]
You are told that the silver electrode is the cathode, reduction is a gain of electrons at the cathode:
Cathode half-reaction: [latex]\scriptsize \displaystyle \text{Ag}_{{\left( {\text{aq}} \right)}}^{\text{+}}\text{+}{{\text{e}}^{\text{-}}}\to \text{A}{{\text{g}}_{{\left( \text{s} \right)}}}[/latex] - [latex]\scriptsize \displaystyle \text{C}{{\text{u}}_{{\left( \text{s} \right)}}}\text{+2Ag}_{{\left( {\text{aq}} \right)}}^{\text{+}}\to \text{Cu}_{{\left( {\text{aq}} \right)}}^{{\text{2+}}}\text{+2A}{{\text{g}}_{{\left( \text{s} \right)}}}[/latex]
OR
[latex]\scriptsize \displaystyle \text{C}{{\text{u}}_{{\left( \text{s} \right)}}}\text{+2AgN}{{\text{O}}_{{\text{3}\left( {\text{aq}} \right)}}}\to \text{Cu}{{\left( {\text{N}{{\text{O}}_{\text{3}}}} \right)}_{{\text{2}\left( {\text{aq}} \right)}}}\text{+2A}{{\text{g}}_{{\left( \text{s} \right)}}}[/latex] - [latex]\scriptsize \displaystyle \text{C}{{\text{u}}_{{\left( \text{s} \right)}}}\left| {\text{Cu}_{{\left( {\text{aq}} \right)}}^{{\text{2+}}}} \right|\left| {\text{Ag}_{{\left( {\text{aq}} \right)}}^{\text{+}}} \right|\text{A}{{\text{g}}_{{\left( \text{s} \right)}}}[/latex]
- Remember that conventional current flow is in the opposite direction to electron flow.
- The copper electrode is the anode, oxidation is a loss of electrons at the anode:
- .
- Electrolysis
- es. Reduction is a gain of electrons. In an electrolytic cell the electrode connected to the negative terminal of the battery is negative. When the metal cations encounter this electrode, they gain electrons and form metal atoms that deposit on the electrode.
- [latex]\scriptsize \displaystyle \text{Ag}_{{\left( {\text{aq}} \right)}}^{\text{+}}\text{+ }{{\text{e}}^{\text{-}}}\to \text{ A}{{\text{g}}_{{\left( \text{s} \right)}}}[/latex]
Exercise 1.4
- .
- The oxidation number of O is [latex]\scriptsize \displaystyle -2[/latex]. O is neither oxidised nor reduced.
The oxidation number of Cu in:
[latex]\scriptsize \displaystyle \begin{align*}&\text{Cu}{{\text{O}}_{{\left( \text{s} \right)}}}\text{ is +2}\\&\text{C}{{\text{u}}_{{\left( \text{s} \right)}}}\text{ is 0}\\&\text{Cu}_{{\left( \text{s} \right)}}^{{\text{2+}}}\text{+2}{{\text{e}}^{\text{-}}}\to \text{C}{{\text{u}}_{{\left( \text{s} \right)}}} \end{align*}[/latex]
[latex]\scriptsize \displaystyle \text{C}{{\text{u}}^{{\text{2+}}}}[/latex] gains two electrons to form Cu. Copper is reduced.
.
The oxidation number of H in:
[latex]\scriptsize \displaystyle \begin{align*}&\text{H}{{\text{2}}_{{\left( \text{g} \right)\text{ }}}}\text{is 0}\\&{{\text{H}}_{\text{2}}}{{\text{O}}_{{\left( \text{g} \right)}}}\text{ is +1}\\&{{\text{H}}_{{\text{2}\left( \text{g} \right)}}}\to \text{2H}_{{\left( \text{g} \right)}}^{\text{+}}\text{+2}{{\text{e}}^{\text{-}}} \end{align*}[/latex]
[latex]\scriptsize \displaystyle {{\text{H}}_{\text{2}}}[/latex] loses two electrons to form [latex]\scriptsize \displaystyle \text{2}{{\text{H}}^{\text{+}}}[/latex]. Hydrogen is oxidised.
Note: Each hydrogen atom in [latex]\scriptsize \displaystyle {{\text{H}}_{\text{2}}}[/latex] releases one electron, and these two electrons are taken up by a [latex]\scriptsize \displaystyle \text{C}{{\text{u}}^{{\text{2+}}}}[/latex] ion. - The oxidation number of O is [latex]\scriptsize \displaystyle -2[/latex]. O is neither oxidised nor reduced.
The oxidation number of N in:
[latex]\scriptsize \displaystyle \begin{align*}&\text{N}{{\text{O}}_{{\left( \text{g} \right)\text{ }}}}\text{is +2}\\&{{\text{N}}_{{\text{2}\left( \text{g} \right)}}}\text{ is 0}\\&\text{2N}_{{\left( \text{g} \right)}}^{{\text{2+}}}\text{+4}{{\text{e}}^{\text{-}}}\to {{\text{N}}_{{\text{2}\left( \text{g} \right)}}} \end{align*}[/latex]
Two [latex]\scriptsize \displaystyle {{\text{N}}^{{\text{2+}}}}[/latex] each gain two electrons to become [latex]\scriptsize \displaystyle {{\text{N}}_{\text{2}}}[/latex]. Nitrogen is reduced.
The oxidation number of C in:
[latex]\scriptsize \displaystyle \begin{align*}&\text{C}{{\text{O}}_{{\left( \text{g} \right)}}}\text{ is +2}\\&\text{C}{{\text{O}}_{{\text{2}\left( \text{g} \right)}}}\text{ is +4}\\&\text{C}_{{\left( \text{g} \right)}}^{{\text{2+}}}\to \text{C}_{{\left( \text{g} \right)}}^{{\text{4+}}}\text{+2}{{\text{e}}^{\text{-}}} \end{align*}[/latex]
[latex]\scriptsize \displaystyle {{\text{C}}^{{\text{2+}}}}[/latex] loses two electrons to form [latex]\scriptsize \displaystyle {{\text{C}}^{{\text{4+}}}}[/latex]. Carbon is oxidised. - The oxidation number of the ion [latex]\scriptsize \displaystyle \text{S}{{\text{O}}_{\text{4}}}\text{ is -2}[/latex] as both a reactant and a product.
The oxidation number of O is [latex]\scriptsize \displaystyle -2[/latex]. O is neither oxidised nor reduced.
The oxidation number of [latex]\scriptsize \displaystyle {{\text{O}}_{\text{4}}}\text{ in SO}_{\text{2}}^{{\text{-4 }}}\text{is -8}[/latex]. Therefore the oxidation number of S in [latex]\scriptsize \displaystyle \text{SO}_{\text{2}}^{{\text{-4}}}[/latex]is[latex]\scriptsize \displaystyle +6[/latex]. S is neither oxidised nor reduced.
.
The oxidation number of Mg in:
[latex]\scriptsize \displaystyle\begin{align*}&\text{M}{{\text{g}}_{{\left( \text{s} \right)}}}\text{ is 0}\\&\text{MgS}{{\text{O}}_{{\text{4}\left( {\text{aq}} \right)}}}\text{ is +2}\\&\text{M}{{\text{g}}_{{\left( \text{s} \right)}}}\to \text{Mg}_{{\left( {\text{aq}} \right)}}^{{\text{2+}}}\text{+2}{{\text{e}}^{\text{-}}} \end{align*}[/latex]
Mg loses two electrons to become [latex]\scriptsize \displaystyle \text{M}{{\text{g}}^{{\text{2+}}}}[/latex]. Magnesium is oxidised.
.
The oxidation number of Fe in:
[latex]\scriptsize \displaystyle \begin{align*}&\text{FeS}{{\text{O}}_{{\text{4}\left( {\text{aq}} \right)}}}\text{ is +2}\\&\text{F}{{\text{e}}_{{\left( \text{s} \right)\text{ }}}}\text{is 0}\\&\text{Fe}_{{\left( {\text{aq}} \right)}}^{{\text{2+}}}\text{+2}{{\text{e}}^{\text{-}}}\to \text{F}{{\text{e}}_{{\left( \text{s} \right)}}} \end{align*}[/latex]
[latex]\scriptsize \displaystyle \text{F}{{\text{e}}^{{\text{2+}}}}[/latex] gains two electrons to become [latex]\scriptsize \displaystyle \text{Fe}\text{.}[/latex] Iron is reduced.
- The oxidation number of O is [latex]\scriptsize \displaystyle -2[/latex]. O is neither oxidised nor reduced.
- .
- [latex]\scriptsize \displaystyle \text{F}{{\text{e}}_{{\left( \text{s} \right)}}}\to \text{Fe}_{{\left( {\text{aq}} \right)}}^{{\text{3+}}}\text{+3}{{\text{e}}^{\text{-}}}[/latex]
- [latex]\scriptsize \displaystyle \text{Fe}_{{\left( {\text{aq}} \right)}}^{{\text{2+}}}\text{+2}{{\text{e}}^{\text{-}}}\to \text{F}{{\text{e}}_{{\left( \text{s} \right)}}}[/latex]
- An oxidising agent causes oxidation and is itself reduced: [latex]\scriptsize \displaystyle \text{Fe}_{{\left( {\text{aq}} \right)}}^{{\text{2+}}}[/latex]
- A reducing agent causes reduction and is itself oxidised: [latex]\scriptsize \displaystyle \text{F}{{\text{e}}_{{\left( \text{s} \right)}}}[/latex]
- Oxidation is loss at the anode, therefore [latex]\scriptsize \displaystyle \text{F}{{\text{e}}_{{\left( \text{s} \right)}}}[/latex] in a oxidation half-reaction is the anode.
Reduction is gain at the cathode, therefore [latex]\scriptsize \displaystyle \text{F}{{\text{e}}_{{\left( \text{s} \right)}}}[/latex] in a reduction half-reaction is the cathode.
[latex]\scriptsize \displaystyle \text{F}{{\text{e}}_{{\left( \text{s} \right)}}}\left| {\text{Fe}_{{\left( {\text{aq}} \right)}}^{{\text{3+}}}} \right|\left| {\text{Fe}_{{\left( {\text{aq}} \right)}}^{{\text{2+}}}} \right|\text{F}{{\text{e}}_{{\left( \text{s} \right)}}}[/latex]
- .
- The cathode is written on the right; therefore manganese is the cathode. Reduction is gain at the cathode.
[latex]\scriptsize \displaystyle \text{Mn}_{{\left( {\text{aq}} \right)}}^{{\text{2+}}}\text{+2}{{\text{e}}^{\text{-}}}\to \text{M}{{\text{n}}_{{\left( \text{s} \right)}}}[/latex]
The anode is written on the left; therefore magnesium is the anode. Oxidation is loss at the anode.
[latex]\scriptsize \displaystyle \text{M}{{\text{g}}_{{\left( \text{s} \right)}}}\to \text{Mg}_{{\left( {\text{aq}} \right)}}^{{\text{2+}}}\text{+2}{{\text{e}}^{\text{-}}}[/latex] - [latex]\scriptsize \displaystyle \text{M}{{\text{g}}_{{\left( \text{s} \right)}}}\text{+Mn}_{{\left( {\text{aq}} \right)}}^{{\text{2+}}}\to \text{Mg}_{{\left( {\text{aq}} \right)}}^{{\text{2+}}}\text{+M}{{\text{n}}_{{\left( \text{s} \right)}}}[/latex]
- Magnesium metal and manganese metal.
- Magnesium sulfate and manganese sulfate, or magnesium nitrate and manganese nitrate
- .
- The cathode is written on the right; therefore manganese is the cathode. Reduction is gain at the cathode.
Exercise 1.5
1. Sacrificial anodes are used to protect metal structures from corroding.
2. Sacrificial anodes work by oxidising more quickly than the metal it is protecting, being consumed completely before the other metal reacts with the electrolytes.
3. Several different forms of cathode protection are forming alloys, plating, and galvanizing the metal.
Unit 1: Assessment
- The unbalanced reduction half-reaction is:
[latex]\scriptsize \displaystyle \text{MnO}_{{\left( {\text{aq}} \right)}}^{{\text{-4}}}\to \text{Mn}_{{\left( {\text{aq}} \right)}}^{{\text{2+}}}[/latex]
As this is in an acid medium, we can add water molecules to the right and [latex]\scriptsize \displaystyle {{\text{H}}^{\text{+}}}[/latex] ions to the left to balance the oxygen and hydrogen atoms:
[latex]\scriptsize \displaystyle \text{MnO}_{{\left( {\text{aq}} \right)}}^{{\text{-4}}}\text{+8}{{\text{H}}^{\text{+}}}\to \text{Mn}_{{\left( {\text{aq}} \right)}}^{{\text{2+}}}\text{+4}{{\text{H}}_{\text{2}}}{{\text{O}}_{{\left( \text{l} \right)}}}[/latex]
Balance the charge by adding five electrons to the left (this makes sense as this is the reduction half-reaction, and [latex]\scriptsize \displaystyle \text{M}{{\text{n}}^{{\text{7+}}}}\to \text{M}{{\text{n}}^{{\text{2+}}}}[/latex]):
[latex]\scriptsize \displaystyle \text{MnO}_{{\left( {\text{aq}} \right)}}^{{\text{-4}}}\text{+8H}_{{\left( {\text{aq}} \right)}}^{\text{+}}\text{+5}{{\text{e}}^{-}}\to \text{Mn}_{{\left( {\text{aq}} \right)}}^{{\text{2+}}}\text{+4}{{\text{H}}_{\text{2}}}{{\text{O}}_{{\left( \text{l} \right)}}}[/latex]
The unbalanced oxidation half-reaction is:
[latex]\scriptsize \displaystyle {{\text{H}}_{\text{2}}}{{\text{O}}_{{\text{2}\left( \text{l} \right)}}}\to {{\text{O}}_{{\text{2}\left( \text{g} \right)}}}[/latex]
Add [latex]\scriptsize \displaystyle {{\text{H}}^{\text{+}}}[/latex] ions to the right to balance the hydrogen atoms:
[latex]\scriptsize \displaystyle {{\text{H}}_{\text{2}}}{{\text{O}}_{{\text{2}\left( \text{l} \right)}}}\to {{\text{O}}_{{\text{2}\left( \text{g} \right)}}}\text{+2H}_{{\left( {\text{aq}} \right)}}^{\text{+}}[/latex]
Balance the charge by adding two electrons to the right (this makes sense as this is the oxidation half-reaction, and [latex]\scriptsize \displaystyle \text{2}{{\text{O}}^{\text{-}}}\to \text{ }{{\text{O}}_{\text{2}}}[/latex]):
[latex]\scriptsize \displaystyle {{\text{H}}_{\text{2}}}{{\text{O}}_{{\text{2}\left( \text{l} \right)}}}\to {{\text{O}}_{{\text{2}\left( \text{g} \right)}}}\text{+2H}_{{\left( {\text{aq}} \right)}}^{\text{+}}\text{+2}{{\text{e}}^{\text{-}}}[/latex]
We multiply the reduction half-reaction by [latex]\scriptsize \displaystyle 2[/latex] and the oxidation half-reaction by [latex]\scriptsize \displaystyle 5[/latex] to balance the number of electrons in both equations:
[latex]\scriptsize \displaystyle \begin{align*}\text{2MnO}_{{\left( {\text{aq}} \right)}}^{{\text{-4}}}\text{+16H}_{{\left( {\text{aq}} \right)}}^{\text{+}}\text{+10}{{\text{e}}^{\text{-}}}&\to \text{2Mn}_{{\left( {\text{aq}} \right)}}^{{\text{2+}}}\text{+8}{{\text{H}}_{\text{2}}}{{\text{O}}_{{\left( \text{l} \right)}}}\\\text{5}{{\text{H}}_{\text{2}}}{{\text{O}}_{{\text{2}\left( \text{l} \right)}}}&\to \text{5}{{\text{O}}_{{\text{2}\left( \text{g} \right)}}}\text{+10H}_{{\left( {\text{aq}} \right)}}^{\text{+}}+\text{10}{{\text{e}}^{\text{-}}} \end{align*}[/latex]
Adding the two equations together gives the balanced equation (electrons are equal on both sides and can be removed):
[latex]\scriptsize \displaystyle \text{2MnO}_{{\left( {\text{aq}} \right)}}^{{\text{-4}}}\text{+16H}_{{\left( {\text{aq}} \right)}}^{\text{+}}\text{+5}{{\text{H}}_{\text{2}}}{{\text{O}}_{{\text{2}\left( \text{l} \right)}}}\to \text{5}{{\text{O}}_{{\text{2}\left( \text{g} \right)}}}\text{+10H}_{{\left( {\text{aq}} \right)}}^{\text{+}}\text{+2Mn}_{{\left( {\text{aq}} \right)}}^{{\text{2+}}}\text{+8}{{\text{H}}_{\text{2}}}{{\text{O}}_{{\left( \text{l} \right)}}}[/latex]
Removing any extra [latex]\scriptsize \displaystyle {{\text{H}}^{\text{+}}}[/latex] ions we get:
[latex]\scriptsize \displaystyle \text{2MnO}_{{\left( {\text{aq}} \right)}}^{{\text{-4}}}\text{+6H}_{{\left( {\text{aq}} \right)}}^{\text{+}}\text{+5}{{\text{H}}_{\text{2}}}{{\text{O}}_{{\text{2}\left( \text{l} \right)}}}\to \text{5}{{\text{O}}_{{\text{2}\left( \text{g} \right)}}}\text{+2Mn}_{{\left( {\text{aq}} \right)}}^{{\text{2+}}}\text{+8}{{\text{H}}_{\text{2}}}{{\text{O}}_{{\left( \text{l} \right)}}}[/latex] - The oxidising agent causes another reactant to be oxidised and is itself reduced.
- [latex]\scriptsize \displaystyle {{\text{H}}^{\text{+}}}[/latex] remains as [latex]\scriptsize \displaystyle {{\text{H}}^{\text{+}}}[/latex] in [latex]\scriptsize \displaystyle {{\text{H}}_{\text{2}}}\text{O}[/latex]
- [latex]\scriptsize \displaystyle \text{C}{{\text{r}}^{{\text{3+}}}}[/latex] is a product.
- [latex]\scriptsize \displaystyle \text{S}{{\text{O}}_{\text{2}}}[/latex] contains [latex]\scriptsize \displaystyle {{\text{S}}^{{\text{4+}}}}[/latex], while [latex]\scriptsize \displaystyle \text{SO}_{\text{2}}^{{\text{-4}}}[/latex] contains [latex]\scriptsize \displaystyle {{\text{S}}^{{\text{6+}}}}[/latex]. Therefore [latex]\scriptsize \displaystyle {{\text{S}}^{{\text{4+}}}}[/latex] loses two electrons and is oxidised.
- [latex]\scriptsize \displaystyle \text{C}{{\text{r}}_{\text{2}}}{{\text{O}}_{\text{2}}}^{{\text{-7}}}[/latex] contains [latex]\scriptsize \displaystyle \text{C}{{\text{r}}^{{\text{6+}}}}[/latex]. Therefore [latex]\scriptsize \displaystyle \text{C}{{\text{r}}^{{\text{6+}}}}[/latex] gains three electrons to become [latex]\scriptsize \displaystyle \text{C}{{\text{r}}^{{\text{3+}}}}[/latex]and is reduced. It is the oxidising agent.
The answer is d. [latex]\scriptsize \displaystyle \text{C}{{\text{r}}_{\text{2}}}{{\text{O}}_{\text{2}}}^{{\text{-7}}}[/latex].
- .
- The cathode is written on the right; therefore silver is the cathode. Reduction is gain at the cathode.
[latex]\scriptsize \displaystyle \text{Ag}_{{\left( {\text{aq}} \right)}}^{\text{+}}\text{+}{{\text{e}}^{\text{-}}}\to \text{A}{{\text{g}}_{{\left( \text{s} \right)}}}[/latex]
The anode is written on the left; therefore tin is the anode. Oxidation is loss at the anode.
[latex]\scriptsize \displaystyle \text{S}{{\text{n}}_{{\left( \text{s} \right)}}}\to \text{Sn}_{{\left( {\text{aq}} \right)}}^{{\text{2+}}}\text{+2}{{\text{e}}^{\text{-}}}[/latex] - To balance the charges the cathode half-reaction must be multiplied by [latex]\scriptsize \displaystyle 2[/latex].
[latex]\scriptsize \displaystyle\begin{align*}\text{2Ag}_{{\left( {\text{aq}} \right)}}^{\text{+}}\text{+2}{{\text{e}}^{\text{-}}}&\to \text{2A}{{\text{g}}_{{\left( \text{s} \right)}}}\\\text{S}{{\text{n}}_{{\left( \text{s} \right)}}}\text{+2Ag}_{{\left( {\text{aq}} \right)}}^{\text{+}}&\to \text{Sn}_{{\left( {\text{aq}} \right)}}^{{\text{2+}}}\text{+2A}{{\text{g}}_{{\left( \text{s} \right)}}} \end{align*}[/latex] - .
- The cathode is written on the right; therefore silver is the cathode. Reduction is gain at the cathode.
- .
- Chlorine is the strongest oxidising agent.
- Calcium is the strongest reducing agent.
- .
- Galvanic cell
- Reduction
- No, a galvanic cell requires two electrodes with different reaction potentials (different metals).
- .
- False. The anode in an electrolytic cell has a positive charge.
- True
- True
- .
- Only (iii) has both oxidation and reduction occurring (i.e. a change in the oxidation numbers).
iii) [latex]\scriptsize \displaystyle \text{2FeC}{{\text{l}}_{{\text{3}\left( \text{s} \right)}}}\text{+2}{{\text{H}}_{\text{2}}}{{\text{O}}_{{\left( \text{l} \right)}}}\text{+S}{{\text{O}}_{{\text{2}\left( {\text{aq}} \right)}}}\to {{\text{H}}_{\text{2}}}\text{S}{{\text{O}}_{{\text{4}\left( {\text{aq}} \right)}}}\text{+2HCl+2FeC}{{\text{l}}_{{\text{2}\left( {\text{aq}} \right)}}}[/latex] - Bromine gains electrons (is reduced), therefore bromine acts as an oxidising agent. Iodide loses electrons (is oxidised), therefore iodide acts as a reducing agent. In the reverse reaction iodine would gain electrons (be reduced) and act as an oxidising agent.
iii) the iodide ions are oxidised. - Loss of electrons is oxidation. The larger, negative value will be oxidised more easily. [latex]\scriptsize \displaystyle \text{Y}\to {{\text{Y}}^{\text{+}}}\text{+}{{\text{e}}^{\text{-}}}[/latex]
iii) Y
- Only (iii) has both oxidation and reduction occurring (i.e. a change in the oxidation numbers).
Media Attributions
- Fig 1 © DHET is licensed under a CC BY (Attribution) license
- Fig 2 © DHET is licensed under a CC BY (Attribution) license
- Fig 3 © DHET is licensed under a CC BY (Attribution) license
- Fig 4 is licensed under a All Rights Reserved license
- Fig 5 © Libretext is licensed under a CC BY-NC-SA (Attribution NonCommercial ShareAlike) license
- Fig 6 © Siyavula is licensed under a CC BY-NC (Attribution NonCommercial) license
- Fig 7 © Siyavula is licensed under a CC BY-NC (Attribution NonCommercial) license
- Fig 8 © Siyavula is licensed under a CC BY-NC (Attribution NonCommercial) license
- Fig 9 © DHET is licensed under a CC BY (Attribution) license
- Fig 10 © Petr Kratochvil is licensed under a CC0 (Creative Commons Zero) license
- Fig 11 © Siyavula is licensed under a CC BY-NC (Attribution NonCommercial) license
- Fig 12 © Siyavula is licensed under a CC BY-NC (Attribution NonCommercial) license
- Fig 13 © DHET is licensed under a CC BY (Attribution) license
- Fig 14 © BatteryGuy is licensed under a CC BY (Attribution) license
- Fig 15 © RSC is licensed under a CC BY (Attribution) license
- Fig 16 © Zwergelstern is licensed under a CC BY-SA (Attribution ShareAlike) license
- Fig 17 © Siyavula is licensed under a CC BY-NC (Attribution NonCommercial) license
- Fig 18 © Siyavula is licensed under a CC BY-NC (Attribution NonCommercial) license
- Fig 19 © Siyavula is licensed under a CC BY-NC (Attribution NonCommercial) license
the loss of electrons during a reaction by a molecule, atom or ion.
a chemical reaction that involves the gaining of electrons by one of the atoms involved in the reaction between two chemicals
a substance that tends to bring about reduction by being oxidized and losing electrons
a substance that tends to bring about oxidation by being reduced and gaining electrons
a method of driving chemical reactions by passing an electric current through an electrolyte
solutions that contain free ions, and which therefore behave as conductors of charges (electrical conductor) in solution
the electrode of an electrochemical cell at which reduction occurs; positive ions form at the cathode
the electrode where an oxidation reaction occurs; at the anode, negative ions form due to its electrical potential
electrical conductors that connect the electrochemical species from its solution to the external electrical circuit of the cell
ions which are not involved or changed in a reaction
an electrochemical cell that converts electrical potential energy to chemical potential energy by using electricity to drive a non-spontaneous chemical reaction
an electrochemical cell which converts chemical potential energy to electrical potential energy through a spontaneous chemical reaction
a structure that consists of a conductive electrode surrounded by a conductive electrolyte
a reaction that involves a transfer of electrons; there is a conversion of chemical potential energy to electrical potential energy, or electrical potential energy to chemical potential energy
a device where electrochemical reactions take place
a material which contains an electrolytic solution and acts as a connection between two half-cells (completes the circuit); it maintains electrical neutrality in the electrolytes in the half-cell compartments
the process of applying a protective layer of zinc on a metal